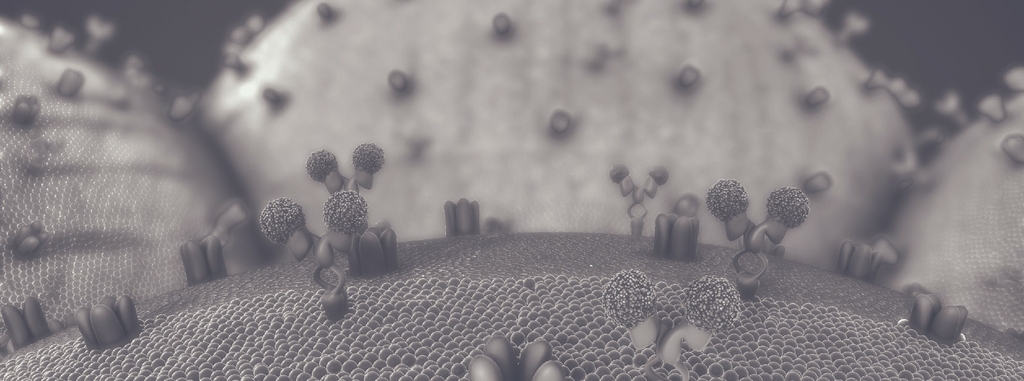
Om te delen moet een cel het ‘warburgmetabolisme’ vertonen. Er wordt meer glucose en glutamine opgenomen, de intracellulaire pH en het natriumgehalte gaan omhoog, de cel beschermt zich intensiever tegen oxidatieve stress en scheidt lactaat uit. Otto Warburg ontdekte zijn warburgeffect bij kanker. Logischerwijs, maar incorrect, dacht hij dat de mitochondriën van kankercellen disfunctioneren. Later bleek het warburgeffect een eigenschap van alle delende cellen. Hierbij worden de metabole wegen die we kennen uit de leerboeken ‘ge-reprogrammeerd’. De intensiteit waarmee de glycolyse, citroenzuurcyclus en de oxidatieve fosforylering worden doorlopen verandert en de cel maakt uit één molecuul glucose niet 36 maar slechts 2 moleculen ATP. Het metabolisme is gericht op het maken van biomassa voor nieuwe cellen, niet op het genereren van de energie die nodig is voor het functioneren van een gedifferentieerde (post-mitotische) eindcel, en zoals dat in de leerboeken staat. Het warburgeffect duikt nu op als een rode draad door ogenschijnlijk uiteenlopende degeneratieve ziektes. De geschiedenis herhaalt zich want de mitochondriën krijgen weer de schuld. Wat heeft kanker gemeen met hart- en nierfalen, epilepsie, neurodegeneratieve ziektes zoals alzheimer en parkinson, het chronische-vermoeidheidssyndroom en een virusinfect? Het antwoord is: verouderde (senescente) cellen.
Beste bezoeker, u heeft geen toegang.
Enkel (web)abonnees hebben toegang tot tijdschriftartikelen. Het webabonnement is nog in de maak.
U kunt zich wel alvast (gratis) registreren en tal van andere webartikelen raadplegen!
Auteur
Trefwoorden:
Verschenen in
Referenties
Warburg effect
1. López-Lázaro M. The warburg effect: why and how do cancer cells activate glycolysis in the presence of oxygen? Anticancer Agents Med Chem. 2008 Apr;8(3):305-12. doi: 10.2174/187152008783961932. PMID: 18393789.
https://pubmed.ncbi.nlm.nih.gov/18393789/
2. Vander Heiden MG, Cantley LC, Thompson CB. Understanding the Warburg effect: the metabolic requirements of cell proliferation. Science. 2009 May 22;324(5930):1029-33. doi: 10.1126/science.1160809. PMID: 19460998; PMCID: PMC2849637.
https://pubmed.ncbi.nlm.nih.gov/19460998/
3. Dang CV. Links between metabolism and cancer. Genes Dev. 2012 May 1;26(9):877-90. doi: 10.1101/gad.189365.112. PMID: 22549953; PMCID: PMC3347786.
https://pubmed.ncbi.nlm.nih.gov/22549953/
4. Ward PS, Thompson CB. Metabolic reprogramming: a cancer hallmark even warburg did not anticipate. Cancer Cell. 2012 Mar 20;21(3):297-308. doi: 10.1016/j.ccr.2012.02.014. PMID: 22439925; PMCID: PMC3311998.
https://pubmed.ncbi.nlm.nih.gov/22439925/
5. Jeoung NH. Pyruvate Dehydrogenase Kinases: Therapeutic Targets for Diabetes and Cancers. Diabetes Metab J. 2015 Jun;39(3):188-97. doi: 10.4093/dmj.2015.39.3.188. PMID: 26124988; PMCID: PMC4483603.
https://pubmed.ncbi.nlm.nih.gov/26124988/
6. Liberti MV, Locasale JW. The Warburg Effect: How Does it Benefit Cancer Cells? Trends Biochem Sci. 2016 Mar;41(3):211-218. doi: 10.1016/j.tibs.2015.12.001. Epub 2016 Jan 5. Erratum in: Trends Biochem Sci. 2016 Mar;41(3):287. Erratum in: Trends Biochem Sci. 2016 Mar;41(3):287. PMID: 26778478; PMCID: PMC4783224.
https://pubmed.ncbi.nlm.nih.gov/26778478/
7. Abdel-Haleem AM, Lewis NE, Jamshidi N, Mineta K, Gao X, Gojobori T. The Emerging Facets of Non-Cancerous Warburg Effect. Front Endocrinol (Lausanne). 2017 Oct 23;8:279. doi: 10.3389/fendo.2017.00279. PMID: 29109698; PMCID: PMC5660072.
https://pubmed.ncbi.nlm.nih.gov/29109698/
8. Burns JS, Manda G. Metabolic Pathways of the Warburg Effect in Health and Disease: Perspectives of Choice, Chain or Chance. Int J Mol Sci. 2017 Dec 19;18(12):2755. doi: 10.3390/ijms18122755. PMID: 29257069; PMCID: PMC5751354.
https://pubmed.ncbi.nlm.nih.gov/29257069/
9. Corbet C. Stem Cell Metabolism in Cancer and Healthy Tissues: Pyruvate in the Limelight. Front Pharmacol. 2018 Jan 4;8:958. doi: 10.3389/fphar.2017.00958. PMID: 29403375; PMCID: PMC5777397.
https://pubmed.ncbi.nlm.nih.gov/29403375/
10. Zangari J, Petrelli F, Maillot B, Martinou JC. The Multifaceted Pyruvate Metabolism: Role of the Mitochondrial Pyruvate Carrier. Biomolecules. 2020 Jul 17;10(7):1068. doi: 10.3390/biom10071068. PMID: 32708919; PMCID: PMC7407832.
https://pubmed.ncbi.nlm.nih.gov/32708919/
11. Samec M, Liskova A, Koklesova L, Samuel SM, Zhai K, Buhrmann C, Varghese E, Abotaleb M, Qaradakhi T, Zulli A, Kello M, Mojzis J, Zubor P, Kwon TK, Shakibaei M, Büsselberg D, Sarria GR, Golubnitschaja O, Kubatka P. Flavonoids against the Warburg phenotype-concepts of predictive, preventive and personalised medicine to cut the Gordian knot of cancer cell metabolism. EPMA J. 2020 Jul 30;11(3):377-398. doi: 10.1007/s13167-020-00217-y. PMID: 32843908; PMCID: PMC7429635.
https://pubmed.ncbi.nlm.nih.gov/32843908/
12. Bouillaud F, Hammad N, Schwartz L. Warburg Effect, Glutamine, Succinate, Alanine, When Oxygen Matters. Biology (Basel). 2021 Oct 4;10(10):1000. doi: 10.3390/biology10101000. PMID: 34681099; PMCID: PMC8533123.
https://pubmed.ncbi.nlm.nih.gov/34681099/
13. Vaupel P, Multhoff G. Revisiting the Warburg effect: historical dogma versus current understanding. J Physiol. 2021 Mar;599(6):1745-1757. doi: 10.1113/JP278810. Epub 2021 Jan 4. PMID: 33347611.
https://pubmed.ncbi.nlm.nih.gov/33347611/
14. Kornberg MD. The immunologic Warburg effect: Evidence and therapeutic opportunities in autoimmunity. Wiley Interdiscip Rev Syst Biol Med. 2020 Sep;12(5):e1486. doi: 10.1002/wsbm.1486. Epub 2020 Feb 27. PMID: 32105390; PMCID: PMC7507184.
https://pubmed.ncbi.nlm.nih.gov/32105390/
15. Palsson-McDermott EM, O'Neill LA. The Warburg effect then and now: from cancer to inflammatory diseases. Bioessays. 2013 Nov;35(11):965-73. doi: 10.1002/bies.201300084. Epub 2013 Sep 20. PMID: 24115022.
https://pubmed.ncbi.nlm.nih.gov/24115022/
Non-cancer Warburg
7. Abdel-Haleem AM, Lewis NE, Jamshidi N, Mineta K, Gao X, Gojobori T. The Emerging Facets of Non-Cancerous Warburg Effect. Front Endocrinol (Lausanne). 2017 Oct 23;8:279. doi: 10.3389/fendo.2017.00279. PMID: 29109698; PMCID: PMC5660072.
16. Chen Z, Liu M, Li L, Chen L. Involvement of the Warburg effect in non-tumor diseases processes. J Cell Physiol. 2018 Apr;233(4):2839-2849. doi: 10.1002/jcp.25998. Epub 2017 Jun 12. PMID: 28488732.
https://pubmed.ncbi.nlm.nih.gov/28488732/
17. Schwartz L, Henry M, Alfarouk KO, Reshkin SJ, Radman M. Metabolic Shifts as the Hallmark of Most Common Diseases: The Quest for the Underlying Unity. Int J Mol Sci. 2021 Apr 12;22(8):3972. doi: 10.3390/ijms22083972. PMID: 33921428; PMCID: PMC8068795.
https://pubmed.ncbi.nlm.nih.gov/33921428/
SGLT2 inhibition/kidney and heart failure
18. Paneni F, Costantino S, Hamdani N. Regression of left ventricular hypertrophy with SGLT2 inhibitors. Eur Heart J. 2020 Sep 21;41(36):3433-3436. doi: 10.1093/eurheartj/ehaa530. PMID: 32620974.
https://pubmed.ncbi.nlm.nih.gov/32620974/
18a. Zelniker TA, Wiviott SD, Raz I, Im K, Goodrich EL, Bonaca MP, Mosenzon O, Kato ET, Cahn A, Furtado RHM, Bhatt DL, Leiter LA, McGuire DK, Wilding JPH, Sabatine MS. SGLT2 inhibitors for primary and secondary prevention of cardiovascular and renal outcomes in type 2 diabetes: a systematic review and meta-analysis of cardiovascular outcome trials. Lancet. 2019 Jan 5;393(10166):31-39. doi: 10.1016/S0140-6736(18)32590-X. Epub 2018 Nov 10. Erratum in: Lancet. 2019 Jan 5;393(10166):30. PMID: 30424892.
https://pubmed.ncbi.nlm.nih.gov/30424892/
19. Kaneto H, Obata A, Kimura T, Shimoda M, Kinoshita T, Matsuoka TA, Kaku K. Unexpected Pleiotropic Effects of SGLT2 Inhibitors: Pearls and Pitfalls of This Novel Antidiabetic Class. Int J Mol Sci. 2021 Mar 17;22(6):3062. doi: 10.3390/ijms22063062. PMID: 33802741; PMCID: PMC8002535.
https://pubmed.ncbi.nlm.nih.gov/33802741/
20. Muskiet MHA, Heerspink HJL, van Raalte DH. SGLT2 inhibitors: expanding their Empire beyond diabetes. Lancet Diabetes Endocrinol. 2021 Feb;9(2):59-61. doi: 10.1016/S2213-8587(20)30428-9. Epub 2020 Dec 22. PMID: 33357504.
https://pubmed.ncbi.nlm.nih.gov/33357504/
21. Paneni F, Costantino S, Hamdani N. Regression of left ventricular hypertrophy with SGLT2 inhibitors. Eur Heart J. 2020 Sep 21;41(36):3433-3436. doi: 10.1093/eurheartj/ehaa530. PMID: 32620974.
https://pubmed.ncbi.nlm.nih.gov/32620974/
22. Salvatore T, Galiero R, Caturano A, Rinaldi L, Di Martino A, Albanese G, Di Salvo J, Epifani R, Marfella R, Docimo G, Lettieri M, Sardu C, Sasso FC. An Overview of the Cardiorenal Protective Mechanisms of SGLT2 Inhibitors. Int J Mol Sci. 2022 Mar 26;23(7):3651. doi: 10.3390/ijms23073651. PMID: 35409011; PMCID: PMC8998569.
https://pubmed.ncbi.nlm.nih.gov/35409011/
23. Uthman L, Baartscheer A, Schumacher CA, Fiolet JWT, Kuschma MC, Hollmann MW, Coronel R, Weber NC, Zuurbier CJ. Direct Cardiac Actions of Sodium Glucose Cotransporter 2 Inhibitors Target Pathogenic Mechanisms Underlying Heart Failure in Diabetic Patients. Front Physiol. 2018 Nov 21;9:1575. doi: 10.3389/fphys.2018.01575. PMID: 30519189; PMCID: PMC6259641.
https://pubmed.ncbi.nlm.nih.gov/30519189/
23a. Perrone-Filardi P, Avogaro A, Bonora E, Colivicchi F, Fioretto P, Maggioni AP, Sesti G, Ferrannini E. Mechanisms linking empagliflozin to cardiovascular and renal protection. Int J Cardiol. 2017 Aug 15;241:450-456. doi: 10.1016/j.ijcard.2017.03.089. Epub 2017 Mar 23. PMID: 28395981.
https://pubmed.ncbi.nlm.nih.gov/28395981/
SGLT2 inhibition and glucose loss
24. Abdul-Ghani MA, Norton L, DeFronzo RA. Renal sodium-glucose cotransporter inhibition in the management of type 2 diabetes mellitus. Am J Physiol Renal Physiol. 2015 Dec 1;309(11):F889-900. doi: 10.1152/ajprenal.00267.2015. Epub 2015 Sep 9. PMID: 26354881; PMCID: PMC4669360.
https://pubmed.ncbi.nlm.nih.gov/26354881/
25. Vallon V, Thomson SC. The tubular hypothesis of nephron filtration and diabetic kidney disease. Nat Rev Nephrol. 2020 Jun;16(6):317-336. doi: 10.1038/s41581-020-0256-y. Epub 2020 Mar 9. PMID: 32152499; PMCID: PMC7242158.
https://pubmed.ncbi.nlm.nih.gov/32152499/
SGLT2 inhibition and ketone formation
26. Evans M, Cogan KE, Egan B. Metabolism of ketone bodies during exercise and training: physiological basis for exogenous supplementation. J Physiol. 2017 May 1;595(9):2857-2871. doi: 10.1113/JP273185. Epub 2016 Dec 7. PMID: 27861911; PMCID: PMC5407977.
https://pubmed.ncbi.nlm.nih.gov/27861911/
27. Polidori D, Iijima H, Goda M, Maruyama N, Inagaki N, Crawford PA. Intra- and inter-subject variability for increases in serum ketone bodies in patients with type 2 diabetes treated with the sodium glucose co-transporter 2 inhibitor canagliflozin. Diabetes Obes Metab. 2018 May;20(5):1321-1326. doi: 10.1111/dom.13224. Epub 2018 Feb 14. PMID: 29341404; PMCID: PMC5947648.
https://pubmed.ncbi.nlm.nih.gov/29341404/
28. Herring RA, Shojaee-Moradie F, Stevenage M, Parsons I, Jackson N, Mendis J, Middleton B, Umpleby AM, Fielding BA, Davies M, Russell-Jones DL. The SGLT2 Inhibitor Dapagliflozin Increases the Oxidation of Ingested Fatty Acids to Ketones in Type 2 Diabetes. Diabetes Care. 2022 Jun 2;45(6):1408-1415. doi: 10.2337/dc21-2043. PMID: 35312749.
https://pubmed.ncbi.nlm.nih.gov/35312749/
Ketones
28a. Cahill GF Jr, Veech RL. Ketoacids? Good medicine? Trans Am Clin Climatol Assoc. 2003;114:149-61; discussion 162-3. PMID: 12813917; PMCID: PMC2194504.
https://pubmed.ncbi.nlm.nih.gov/12813917/
28b. Veech RL. The therapeutic implications of ketone bodies: the effects of ketone bodies in pathological conditions: ketosis, ketogenic diet, redox states, insulin resistance, and mitochondrial metabolism. Prostaglandins Leukot Essent Fatty Acids. 2004 Mar;70(3):309-19. doi: 10.1016/j.plefa.2003.09.007. PMID: 14769489.
https://pubmed.ncbi.nlm.nih.gov/14769489/
28c. Cahill GF Jr. Fuel metabolism in starvation. Annu Rev Nutr. 2006;26:1-22. doi: 10.1146/annurev.nutr.26.061505.111258. PMID: 16848698.
https://pubmed.ncbi.nlm.nih.gov/16848698/
28d. Kolwicz SC Jr. Ketone Body Metabolism in the Ischemic Heart. Front Cardiovasc Med. 2021 Dec 7;8:789458. doi: 10.3389/fcvm.2021.789458. PMID: 34950719; PMCID: PMC8688810.
https://pubmed.ncbi.nlm.nih.gov/34950719/
28e. Fukao T, Mitchell G, Sass JO, Hori T, Orii K, Aoyama Y. Ketone body metabolism and its defects. J Inherit Metab Dis. 2014 Jul;37(4):541-51. doi: 10.1007/s10545-014-9704-9. Epub 2014 Apr 8. PMID: 24706027.
https://pubmed.ncbi.nlm.nih.gov/24706027/
29. Musso G, Saba F, Cassader M, Gambino R. Diabetic ketoacidosis with SGLT2 inhibitors. BMJ. 2020 Nov 12;371:m4147. doi: 10.1136/bmj.m4147. PMID: 33184044.
https://pubmed.ncbi.nlm.nih.gov/33184044/
29a. Morita M, Kanasaki K. Sodium-glucose cotransporter-2 inhibitors for diabetic kidney disease: Targeting Warburg effects in proximal tubular cells. Diabetes Metab. 2020 Oct;46(5):353-361. doi: 10.1016/j.diabet.2020.06.005. Epub 2020 Sep 3. PMID: 32891754.
https://pubmed.ncbi.nlm.nih.gov/32891754/
Hyperplasia vs. hypertrophy
30. Taylor NA, Wilkinson JG. Exercise-induced skeletal muscle growth. Hypertrophy or hyperplasia? Sports Med. 1986 May-Jun;3(3):190-200. doi: 10.2165/00007256-198603030-00003. PMID: 3520748.
https://pubmed.ncbi.nlm.nih.gov/3520748/
31. Goss RJ. Hypertrophy versus hyperplasia. Science. 1966 Sep 30;153(3744):1615-20. doi: 10.1126/science.153.3744.1615. PMID: 5917072.
https://pubmed.ncbi.nlm.nih.gov/5917072/
32. Pathology. Chapter 1. Cellular Pathology. Physiological and pathological hyperplasia and hypertrophy. Accessed 19 06 2022
https://accessmedicine.mhmedical.com/content.aspx?bookid=499§ionid=4...
32a. Icard P, Fournel L, Wu Z, Alifano M, Lincet H. Interconnection between Metabolism and Cell Cycle in Cancer. Trends Biochem Sci. 2019 Jun;44(6):490-501. doi: 10.1016/j.tibs.2018.12.007. Epub 2019 Jan 14. PMID: 30655165.
https://pubmed.ncbi.nlm.nih.gov/30655165/
33. Alicic RZ, Rooney MT, Tuttle KR. Diabetic Kidney Disease: Challenges, Progress, and Possibilities. Clin J Am Soc Nephrol. 2017 Dec 7;12(12):2032-2045. doi: 10.2215/CJN.11491116. Epub 2017 May 18. PMID: 28522654; PMCID: PMC5718284.
https://pubmed.ncbi.nlm.nih.gov/28522654/
34. Evangelista I, Nuti R, Picchioni T, Dotta F, Palazzuoli A. Molecular Dysfunction and Phenotypic Derangement in Diabetic Cardiomyopathy. Int J Mol Sci. 2019 Jul 2;20(13):3264. doi: 10.3390/ijms20133264. PMID: 31269778; PMCID: PMC6651260.
https://pubmed.ncbi.nlm.nih.gov/31269778/
35. Tan Y, Zhang Z, Zheng C, Wintergerst KA, Keller BB, Cai L. Mechanisms of diabetic cardiomyopathy and potential therapeutic strategies: preclinical and clinical evidence. Nat Rev Cardiol. 2020 Sep;17(9):585-607. doi: 10.1038/s41569-020-0339-2. Epub 2020 Feb 20. PMID: 32080423; PMCID: PMC7849055.
https://pubmed.ncbi.nlm.nih.gov/32080423/
35a. Schmitt R, Melk A. Molecular mechanisms of renal aging. Kidney Int. 2017 Sep;92(3):569-579. doi: 10.1016/j.kint.2017.02.036. Epub 2017 Jul 18. PMID: 28729036.
https://pubmed.ncbi.nlm.nih.gov/28729036/
36. Nakamura M, Sadoshima J. Mechanisms of physiological and pathological cardiac hypertrophy. Nat Rev Cardiol. 2018 Jul;15(7):387-407. doi: 10.1038/s41569-018-0007-y. PMID: 29674714.
https://pubmed.ncbi.nlm.nih.gov/29674714/
Frozen in Warburg effect
37. Wolf G. Cell cycle regulation in diabetic nephropathy. Kidney Int Suppl. 2000 Sep;77:S59-66. doi: 10.1046/j.1523-1755.2000.07710.x. PMID: 10997692.
https://pubmed.ncbi.nlm.nih.gov/10997692/
37a. Wolf G, Sharma K, Chen Y, Ericksen M, Ziyadeh FN. High glucose-induced proliferation in mesangial cells is reversed by autocrine TGF-beta. Kidney Int. 1992 Sep;42(3):647-56. doi: 10.1038/ki.1992.330. PMID: 1357223.
https://pubmed.ncbi.nlm.nih.gov/1357223/
37b. Bishop SP, Zhou Y, Nakada Y, Zhang J. Changes in Cardiomyocyte Cell Cycle and Hypertrophic Growth During Fetal to Adult in Mammals. J Am Heart Assoc. 2021 Jan 19;10(2):e017839. doi: 10.1161/JAHA.120.017839. Epub 2021 Jan 5. PMID: 33399005; PMCID: PMC7955297.
https://pubmed.ncbi.nlm.nih.gov/33399005/
37c. Brooks G, Poolman RA, Li JM. Arresting developments in the cardiac myocyte cell cycle: role of cyclin-dependent kinase inhibitors. Cardiovasc Res. 1998 Aug;39(2):301-11. doi: 10.1016/s0008-6363(98)00125-4. PMID: 9798515.
https://pubmed.ncbi.nlm.nih.gov/9798515/
38. Vallon V, Thomson SC. The tubular hypothesis of nephron filtration and diabetic kidney disease. Nat Rev Nephrol. 2020 Jun;16(6):317-336. doi: 10.1038/s41581-020-0256-y. Epub 2020 Mar 9. PMID: 32152499; PMCID: PMC7242158.
https://pubmed.ncbi.nlm.nih.gov/32152499/
38a. Kim MN, Moon JH, Cho YM. Sodium-glucose cotransporter-2 inhibition reduces cellular senescence in the diabetic kidney by promoting ketone body-induced NRF2 activation. Diabetes Obes Metab. 2021 Nov;23(11):2561-2571. doi: 10.1111/dom.14503. Epub 2021 Aug 10. PMID: 34318973.
https://pubmed.ncbi.nlm.nih.gov/34318973/
39. Uehara-Watanabe N, Okuno-Ozeki N, Minamida A, Nakamura I, Nakata T, Nakai K, Yagi-Tomita A, Ida T, Ikeda K, Kitani T, Yamashita N, Kamezaki M, Kirita Y, Matoba S, Tamagaki K, Kusaba T. Direct evidence of proximal tubular proliferation in early diabetic nephropathy. Sci Rep. 2022 Jan 17;12(1):778. doi: 10.1038/s41598-022-04880-1. PMID: 35039597; PMCID: PMC8763925.
https://pubmed.ncbi.nlm.nih.gov/35039597/
40. Zhang G, Darshi M, Sharma K. The Warburg Effect in Diabetic Kidney Disease. Semin Nephrol. 2018 Mar;38(2):111-120. doi: 10.1016/j.semnephrol.2018.01.002. PMID: 29602394; PMCID: PMC5973839.
https://pubmed.ncbi.nlm.nih.gov/29602394/
41. Verbrugge SAJ, Gehlert S, Stadhouders LEM, Jacko D, Aussieker T, M J de Wit G, Vogel ISP, Offringa C, Schönfelder M, Jaspers RT, Wackerhage H. PKM2 Determines Myofiber Hypertrophy In Vitro and Increases in Response to Resistance Exercise in Human Skeletal Muscle. Int J Mol Sci. 2020 Sep 25;21(19):7062. doi: 10.3390/ijms21197062. PMID: 32992783; PMCID: PMC7583908.
https://pubmed.ncbi.nlm.nih.gov/32992783/
42. Fernandez-Caggiano M, Kamynina A, Francois AA, Prysyazhna O, Eykyn TR, Krasemann S, Crespo-Leiro MG, Vieites MG, Bianchi K, Morales V, Domenech N, Eaton P. Mitochondrial pyruvate carrier abundance mediates pathological cardiac hypertrophy. Nat Metab. 2020 Nov;2(11):1223-1231. doi: 10.1038/s42255-020-00276-5. Epub 2020 Oct 26. PMID: 33106688; PMCID: PMC7610404.
https://pubmed.ncbi.nlm.nih.gov/33106688/
43. Cluntun AA, Badolia R, Lettlova S, Parnell KM, Shankar TS, Diakos NA, Olson KA, Taleb I, Tatum SM, Berg JA, Cunningham CN, Van Ry T, Bott AJ, Krokidi AT, Fogarty S, Skedros S, Swiatek WI, Yu X, Luo B, Merx S, Navankasattusas S, Cox JE, Ducker GS, Holland WL, McKellar SH, Rutter J, Drakos SG. The pyruvate-lactate axis modulates cardiac hypertrophy and heart failure. Cell Metab. 2021 Mar 2;33(3):629-648.e10. doi: 10.1016/j.cmet.2020.12.003. Epub 2020 Dec 16. PMID: 33333007; PMCID: PMC7933116.
https://pubmed.ncbi.nlm.nih.gov/33333007/
44. Kuspriyanti NP, Ariyanto EF, Syamsunarno MRRA. Role of Warburg Effect in Cardiovascular Diseases: A Potential Treatment Option. March 2021, The Open Cardiovascular Medicine Journal 15(1):6-17
https://opencardiovascularmedicinejournal.com/VOLUME/15/PAGE/6/ABSTRACT/
44a. Bertelli PM, Pedrini E, Hughes D, McDonnell S, Pathak V, Peixoto E, Guduric-Fuchs J, Stitt AW, Medina RJ. Long term high glucose exposure induces premature senescence in retinal endothelial cells. Front Physiol. 2022 Aug 26;13:929118. doi: 10.3389/fphys.2022.929118. PMID: 36091370; PMCID: PMC9459081.
https://pubmed.ncbi.nlm.nih.gov/36091370/
44b. Xue WJ, He CF, Zhou RY, Xu XD, Xiang LX, Wang JT, Wang XR, Zhou HG, Guo JC. High glucose and palmitic acid induces neuronal senescence by NRSF/REST elevation and the subsequent mTOR-related autophagy suppression. Mol Brain. 2022 Jul 18;15(1):61. doi: 10.1186/s13041-022-00947-2. PMID: 35850767; PMCID: PMC9290252.
https://pubmed.ncbi.nlm.nih.gov/35850767/
Ketones fill the glucose-energy gap, reducing risk of kidney and heart failure
45. Grabacka M, Pierzchalska M, Dean M, Reiss K. Regulation of Ketone Body Metabolism and the Role of PPARα. Int J Mol Sci. 2016 Dec 13;17(12):2093. doi: 10.3390/ijms17122093. PMID: 27983603; PMCID: PMC5187893.
https://pubmed.ncbi.nlm.nih.gov/27983603/
46. Mudaliar S, Alloju S, Henry RR. Can a Shift in Fuel Energetics Explain the Beneficial Cardiorenal Outcomes in the EMPA-REG OUTCOME Study? A Unifying Hypothesis. Diabetes Care. 2016 Jul;39(7):1115-22. doi: 10.2337/dc16-0542. PMID: 27289124.
https://pubmed.ncbi.nlm.nih.gov/27289124/
47. Santos-Gallego, C.G.; Ibanez, J.A.R.; San Antonio, R.; Ishikawa, K.; Watanabe, S.; Botija, M.B.P.; Sanz Salvo, A.J.; Hajjar, R.; Fuster, V.; Badimon, J. Empagliflozin induces a myocardial metabolic shift from glucose consumption to ketone metabolism that mitigates adverse cardiac remodeling and improves myocardial contractility. J. Am. Coll. Cardiol. 2018, 71, A674
https://reader.elsevier.com/reader/sd/pii/S0735109718312154?token=529E79...
48. McCommis KS, Kovacs A, Weinheimer CJ, Shew TM, Koves TR, Ilkayeva OR, Kamm DR, Pyles KD, King MT, Veech RL, DeBosch BJ, Muoio DM, Gross RW, Finck BN. Nutritional modulation of heart failure in mitochondrial pyruvate carrier-deficient mice. Nat Metab. 2020 Nov;2(11):1232-1247. doi: 10.1038/s42255-020-00296-1. Epub 2020 Oct 26. PMID: 33106690; PMCID: PMC7957960.
https://pubmed.ncbi.nlm.nih.gov/33106690/
49. Ekanayake P, Hupfeld C, Mudaliar S. Sodium-Glucose Cotransporter Type 2 (SGLT-2) Inhibitors and Ketogenesis: the Good and the Bad. Curr Diab Rep. 2020 Nov 23;20(12):74. doi: 10.1007/s11892-020-01359-z. PMID: 33230620.
https://pubmed.ncbi.nlm.nih.gov/33230620/
50. Yurista SR, Chong CR, Badimon JJ, Kelly DP, de Boer RA, Westenbrink BD. Therapeutic Potential of Ketone Bodies for Patients With Cardiovascular Disease: JACC State-of-the-Art Review. J Am Coll Cardiol. 2021 Apr 6;77(13):1660-1669. doi: 10.1016/j.jacc.2020.12.065. Epub 2021 Feb 23. PMID: 33637354.
https://pubmed.ncbi.nlm.nih.gov/33637354/
51. Mollace V, Rosano GMC, Anker SD, Coats AJS, Seferovic P, Mollace R, Tavernese A, Gliozzi M, Musolino V, Carresi C, Maiuolo J, Macrì R, Bosco F, Chiocchi M, Romeo F, Metra M, Volterrani M. Pathophysiological Basis for Nutraceutical Supplementation in Heart Failure: A Comprehensive Review. Nutrients. 2021 Jan 17;13(1):257. doi: 10.3390/nu13010257. PMID: 33477388; PMCID: PMC7829856.
https://pubmed.ncbi.nlm.nih.gov/33477388/
52. Saucedo-Orozco H, Voorrips SN, Yurista SR, de Boer RA, Westenbrink BD. SGLT2 Inhibitors and Ketone Metabolism in Heart Failure. J Lipid Atheroscler. 2022 Jan;11(1):1-19. doi: 10.12997/jla.2022.11.1.1. Epub 2022 Jan 13. PMID: 35118019; PMCID: PMC8792821.
https://pubmed.ncbi.nlm.nih.gov/35118019/
53. Ekanayake P, Mudaliar S. A novel hypothesis linking low-grade ketonaemia to cardio-renal benefits with sodium-glucose cotransporter-2 inhibitors. Diabetes Obes Metab. 2022 Jan;24(1):3-11. doi: 10.1111/dom.14562. Epub 2021 Oct 21. PMID: 34605129.
https://pubmed.ncbi.nlm.nih.gov/34605129/
Ketogenic diet
54. Kolb H, Kempf K, Röhling M, Lenzen-Schulte M, Schloot NC, Martin S. Ketone bodies: from enemy to friend and guardian angel. BMC Med. 2021 Dec 9;19(1):313. doi: 10.1186/s12916-021-02185-0. PMID: 34879839; PMCID: PMC8656040.
https://pubmed.ncbi.nlm.nih.gov/34879839/
55. Zhang W, Guo X, Chen L, Chen T, Yu J, Wu C, Zheng J. Ketogenic Diets and Cardio-Metabolic Diseases. Front Endocrinol (Lausanne). 2021 Nov 2;12:753039. doi: 10.3389/fendo.2021.753039. PMID: 34795641; PMCID: PMC8594484.
https://pubmed.ncbi.nlm.nih.gov/34795641/
56. Ströhle A, Hahn A. Diets of modern hunter-gatherers vary substantially in their carbohydrate content depending on ecoenvironments: results from an ethnographic analysis. Nutr Res. 2011 Jun;31(6):429-35. doi: 10.1016/j.nutres.2011.05.003. Epub 2011 Jun 12. PMID: 21745624.
https://pubmed.ncbi.nlm.nih.gov/21745624/
57. Crosby L, Davis B, Joshi S, Jardine M, Paul J, Neola M, Barnard ND. Ketogenic Diets and Chronic Disease: Weighing the Benefits Against the Risks. Front Nutr. 2021 Jul 16;8:702802. doi: 10.3389/fnut.2021.702802. PMID: 34336911; PMCID: PMC8322232.
https://pubmed.ncbi.nlm.nih.gov/34336911/
57a. Pruimboom L, Muskiet FAJ. Intermittent living; the use of ancient challenges as a vaccine against the deleterious effects of modern life - A hypothesis. Med Hypotheses. 2018 Nov;120:28-42. doi: 10.1016/j.mehy.2018.08.002. Epub 2018 Aug 9. PMID: 30220336.
https://pubmed.ncbi.nlm.nih.gov/30220336/
58. Zhu H, Bi D, Zhang Y, Kong C, Du J, Wu X, Wei Q, Qin H. Ketogenic diet for human diseases: the underlying mechanisms and potential for clinical implementations. Signal Transduct Target Ther. 2022 Jan 17;7(1):11. doi: 10.1038/s41392-021-00831-w. PMID: 35034957; PMCID: PMC8761750.
https://pubmed.ncbi.nlm.nih.gov/35034957/
Adverse effects ketogenic diet
58a. Sampath A, Kossoff EH, Furth SL, Pyzik PL, Vining EP. Kidney stones and the ketogenic diet: risk factors and prevention. J Child Neurol. 2007 Apr;22(4):375-8. doi: 10.1177/0883073807301926. PMID: 17621514.
https://pubmed.ncbi.nlm.nih.gov/17621514/
58b. Bergqvist AG. Long-term monitoring of the ketogenic diet: Do's and Don'ts. Epilepsy Res. 2012 Jul;100(3):261-6. doi: 10.1016/j.eplepsyres.2011.05.020. Epub 2011 Aug 19. PMID: 21855296.
https://pubmed.ncbi.nlm.nih.gov/21855296/
58c. Draaisma JMT, Hampsink BM, Janssen M, van Houdt NBM, Linders ETAM, Willemsen MA. The Ketogenic Diet and Its Effect on Bone Mineral Density: A Retrospective Observational Cohort Study. Neuropediatrics. 2019 Dec;50(6):353-358. doi: 10.1055/s-0039-1693059. Epub 2019 Aug 9. PMID: 31398763.
https://pubmed.ncbi.nlm.nih.gov/31398763/
58d. Bergqvist AG, Schall JI, Stallings VA, Zemel BS. Progressive bone mineral content loss in children with intractable epilepsy treated with the ketogenic diet. Am J Clin Nutr. 2008 Dec;88(6):1678-84. doi: 10.3945/ajcn.2008.26099. PMID: 19064531.
https://pubmed.ncbi.nlm.nih.gov/19064531/
58d. Simm PJ, Bicknell-Royle J, Lawrie J, Nation J, Draffin K, Stewart KG, Cameron FJ, Scheffer IE, Mackay MT. The effect of the ketogenic diet on the developing skeleton. Epilepsy Res. 2017 Oct;136:62-66. doi: 10.1016/j.eplepsyres.2017.07.014. Epub 2017 Jul 26. PMID: 28778055.
https://pubmed.ncbi.nlm.nih.gov/28778055/
58e. Ding J, Xu X, Wu X, Huang Z, Kong G, Liu J, Huang Z, Liu Q, Li R, Yang Z, Liu Y, Zhu Q. Bone loss and biomechanical reduction of appendicular and axial bones under ketogenic diet in rats. Exp Ther Med. 2019 Apr;17(4):2503-2510. doi: 10.3892/etm.2019.7241. Epub 2019 Feb 4. PMID: 30906438; PMCID: PMC6425126.
https://pubmed.ncbi.nlm.nih.gov/30906438/
58f. Acharya P, Acharya C, Thongprayoon C, Hansrivijit P, Kanduri SR, Kovvuru K, Medaura J, Vaitla P, Garcia Anton DF, Mekraksakit P, Pattharanitima P, Bathini T, Cheungpasitporn W. Incidence and Characteristics of Kidney Stones in Patients on Ketogenic Diet: A Systematic Review and Meta-Analysis. Diseases. 2021 May 25;9(2):39. doi: 10.3390/diseases9020039. PMID: 34070285; PMCID: PMC8161846.
https://pubmed.ncbi.nlm.nih.gov/34070285/
58g. Heikura IA, Burke LM, Hawley JA, Ross ML, Garvican-Lewis L, Sharma AP, McKay AKA, Leckey JJ, Welvaert M, McCall L, Ackerman KE. A Short-Term Ketogenic Diet Impairs Markers of Bone Health in Response to Exercise. Front Endocrinol (Lausanne). 2020 Jan 21;10:880. doi: 10.3389/fendo.2019.00880. PMID: 32038477; PMCID: PMC6985427.
https://pubmed.ncbi.nlm.nih.gov/32038477/
58h. Frassetto L, Morris RC Jr, Sellmeyer DE, Todd K, Sebastian A. Diet, evolution and aging--the pathophysiologic effects of the post-agricultural inversion of the potassium-to-sodium and base-to-chloride ratios in the human diet. Eur J Nutr. 2001 Oct;40(5):200-13. doi: 10.1007/s394-001-8347-4. PMID: 11842945.
https://pubmed.ncbi.nlm.nih.gov/11842945/
58i. Sebastian A, Frassetto LA, Sellmeyer DE, Merriam RL, Morris RC Jr. Estimation of the net acid load of the diet of ancestral preagricultural Homo sapiens and their hominid ancestors. Am J Clin Nutr. 2002 Dec;76(6):1308-16. doi: 10.1093/ajcn/76.6.1308. PMID: 12450898.
https://pubmed.ncbi.nlm.nih.gov/12450898/
58j. Adeva MM, Souto G. Diet-induced metabolic acidosis. Clin Nutr. 2011 Aug;30(4):416-21. doi: 10.1016/j.clnu.2011.03.008. Epub 2011 Apr 9. PMID: 21481501.
https://pubmed.ncbi.nlm.nih.gov/21481501/
58k. Carnauba RA, Baptistella AB, Paschoal V, Hübscher GH. Diet-Induced Low-Grade Metabolic Acidosis and Clinical Outcomes: A Review. Nutrients. 2017 May 25;9(6):538. doi: 10.3390/nu9060538. PMID: 28587067; PMCID: PMC5490517.
https://pubmed.ncbi.nlm.nih.gov/28587067/
58l. DiNicolantonio JJ, O'Keefe J. Low-grade metabolic acidosis as a driver of chronic disease: a 21st century public health crisis. Open Heart. 2021 Oct;8(2):e001730. doi: 10.1136/openhrt-2021-001730. PMID: 34702776; PMCID: PMC8549658.
https://pubmed.ncbi.nlm.nih.gov/34702776/
58m. DiNicolantonio JJ, O'Keefe JH. Low-grade metabolic acidosis as a driver of insulin resistance. Open Heart. 2021 Sep;8(2):e001788. doi: 10.1136/openhrt-2021-001788. PMID: 34497064; PMCID: PMC8438953.
https://pubmed.ncbi.nlm.nih.gov/34497064/
58m. Green J, Kleeman CR. Role of bone in regulation of systemic acid-base balance. Kidney Int. 1991 Jan;39(1):9-26. doi: 10.1038/ki.1991.2. PMID: 1706001.
https://pubmed.ncbi.nlm.nih.gov/1706001/
Ketones from medium chain triglycerides (MCT)
59. Lin TY, Liu HW, Hung TM. The Ketogenic Effect of Medium-Chain Triacylglycerides. Front Nutr. 2021 Nov 18;8:747284. doi: 10.3389/fnut.2021.747284. PMID: 34888335; PMCID: PMC8650700.
https://pubmed.ncbi.nlm.nih.gov/34888335/
60. Vandenberghe C, St-Pierre V, Pierotti T, Fortier M, Castellano CA, Cunnane SC. Tricaprylin Alone Increases Plasma Ketone Response More Than Coconut Oil or Other Medium-Chain Triglycerides: An Acute Crossover Study in Healthy Adults. Curr Dev Nutr. 2017 Mar 22;1(4):e000257. doi: 10.3945/cdn.116.000257. PMID: 29955698; PMCID: PMC5998344.
https://pubmed.ncbi.nlm.nih.gov/29955698/
Ketone drink
61. Stubbs BJ, Cox PJ, Evans RD, Santer P, Miller JJ, Faull OK, Magor-Elliott S, Hiyama S, Stirling M, Clarke K. On the Metabolism of Exogenous Ketones in Humans. Front Physiol. 2017 Oct 30;8:848. doi: 10.3389/fphys.2017.00848. PMID: 29163194; PMCID: PMC5670148.
https://pubmed.ncbi.nlm.nih.gov/29163194/
Epilepsy
62. Kim DY, Rho JM. The ketogenic diet and epilepsy. Curr Opin Clin Nutr Metab Care. 2008 Mar;11(2):113-20. doi: 10.1097/MCO.0b013e3282f44c06. PMID: 18301085.
https://pubmed.ncbi.nlm.nih.gov/18301085/
63. Liu YM, Wang HS. Medium-chain triglyceride ketogenic diet, an effective treatment for drug-resistant epilepsy and a comparison with other ketogenic diets. Biomed J. 2013 Jan-Feb;36(1):9-15. doi: 10.4103/2319-4170.107154. PMID: 23515148.
https://pubmed.ncbi.nlm.nih.gov/23515148/
64. Nei M, Ngo L, Sirven JI, Sperling MR. Ketogenic diet in adolescents and adults with epilepsy. Seizure. 2014 Jun;23(6):439-42. doi: 10.1016/j.seizure.2014.02.015. Epub 2014 Mar 12. PMID: 24675110.
https://pubmed.ncbi.nlm.nih.gov/24675110/
65. Kossoff EH, Zupec-Kania BA, Auvin S, Ballaban-Gil KR, Christina Bergqvist AG, Blackford R, Buchhalter JR, Caraballo RH, Cross JH, Dahlin MG, Donner EJ, Guzel O, Jehle RS, Klepper J, Kang HC, Lambrechts DA, Liu YMC, Nathan JK, Nordli DR Jr, Pfeifer HH, Rho JM, Scheffer IE, Sharma S, Stafstrom CE, Thiele EA, Turner Z, Vaccarezza MM, van der Louw EJTM, Veggiotti P, Wheless JW, Wirrell EC; Charlie Foundation; Matthew's Friends; Practice Committee of the Child Neurology Society. Optimal clinical management of children receiving dietary therapies for epilepsy: Updated recommendations of the International Ketogenic Diet Study Group. Epilepsia Open. 2018 May 21;3(2):175-192. doi: 10.1002/epi4.12225. PMID: 29881797; PMCID: PMC5983110.
https://pubmed.ncbi.nlm.nih.gov/29881797/
66. D'Andrea Meira I, Romão TT, Pires do Prado HJ, Krüger LT, Pires MEP, da Conceição PO. Ketogenic Diet and Epilepsy: What We Know So Far. Front Neurosci. 2019 Jan 29;13:5. doi: 10.3389/fnins.2019.00005. PMID: 30760973; PMCID: PMC6361831.
https://pubmed.ncbi.nlm.nih.gov/30760973/
67. Martin-McGill KJ, Bresnahan R, Levy RG, Cooper PN. Ketogenic diets for drug-resistant epilepsy. Cochrane Database Syst Rev. 2020 Jun 24;6(6):CD001903. doi: 10.1002/14651858.CD001903.pub5. PMID: 32588435; PMCID: PMC7387249.
https://pubmed.ncbi.nlm.nih.gov/32588435/
Parkinson, Alzheimer/ketones
68. Croteau E, Castellano CA, Richard MA, Fortier M, Nugent S, Lepage M, Duchesne S, Whittingstall K, Turcotte ÉE, Bocti C, Fülöp T, Cunnane SC. Ketogenic Medium Chain Triglycerides Increase Brain Energy Metabolism in Alzheimer's Disease. J Alzheimers Dis. 2018;64(2):551-561. doi: 10.3233/JAD-180202. PMID: 29914035.
https://pubmed.ncbi.nlm.nih.gov/29914035/
69. Torosyan N, Sethanandha C, Grill JD, Dilley ML, Lee J, Cummings JL, Ossinalde C, Silverman DH. Changes in regional cerebral blood flow associated with a 45 day course of the ketogenic agent, caprylidene, in patients with mild to moderate Alzheimer's disease: Results of a randomized, double-blinded, pilot study. Exp Gerontol. 2018 Oct 1;111:118-121. doi: 10.1016/j.exger.2018.07.009. Epub 2018 Jul 10. PMID: 30006299.
https://pubmed.ncbi.nlm.nih.gov/30006299/
70. Phillips MCL, Murtagh DKJ, Gilbertson LJ, Asztely FJS, Lynch CDP. Low-fat versus ketogenic diet in Parkinson's disease: A pilot randomized controlled trial. Mov Disord. 2018 Aug;33(8):1306-1314. doi: 10.1002/mds.27390. Epub 2018 Aug 11. Erratum in: Mov Disord. 2019 Jan;34(1):157. PMID: 30098269; PMCID: PMC6175383.
https://pubmed.ncbi.nlm.nih.gov/30098269/
71. Fortier M, Castellano CA, Croteau E, Langlois F, Bocti C, St-Pierre V, Vandenberghe C, Bernier M, Roy M, Descoteaux M, Whittingstall K, Lepage M, Turcotte ÉE, Fulop T, Cunnane SC. A ketogenic drink improves brain energy and some measures of cognition in mild cognitive impairment. Alzheimers Dement. 2019 May;15(5):625-634. doi: 10.1016/j.jalz.2018.12.017. Epub 2019 Apr 23. PMID: 31027873.
https://pubmed.ncbi.nlm.nih.gov/31027873/
72. Jensen NJ, Wodschow HZ, Nilsson M, Rungby J. Effects of Ketone Bodies on Brain Metabolism and Function in Neurodegenerative Diseases. Int J Mol Sci. 2020 Nov 20;21(22):8767. doi: 10.3390/ijms21228767. PMID: 33233502; PMCID: PMC7699472.
https://pubmed.ncbi.nlm.nih.gov/33233502/
73. Phillips MCL, Deprez LM, Mortimer GMN, Murtagh DKJ, McCoy S, Mylchreest R, Gilbertson LJ, Clark KM, Simpson PV, McManus EJ, Oh JE, Yadavaraj S, King VM, Pillai A, Romero-Ferrando B, Brinkhuis M, Copeland BM, Samad S, Liao S, Schepel JAC. Randomized crossover trial of a modified ketogenic diet in Alzheimer's disease. Alzheimers Res Ther. 2021 Feb 23;13(1):51. doi: 10.1186/s13195-021-00783-x. PMID: 33622392; PMCID: PMC7901512.
https://pubmed.ncbi.nlm.nih.gov/33622392/
74. Kovács Z, Brunner B, Ari C. Beneficial Effects of Exogenous Ketogenic Supplements on Aging Processes and Age-Related Neurodegenerative Diseases. Nutrients. 2021 Jun 26;13(7):2197. doi: 10.3390/nu13072197. PMID: 34206738; PMCID: PMC8308443.
https://pubmed.ncbi.nlm.nih.gov/34206738/
75. Pietrzak D, Kasperek K, Rękawek P, Piątkowska-Chmiel I. The Therapeutic Role of Ketogenic Diet in Neurological Disorders. Nutrients. 2022 May 6;14(9):1952. doi: 10.3390/nu14091952. PMID: 35565918; PMCID: PMC9102882.
https://pubmed.ncbi.nlm.nih.gov/35565918/
76. Butterfield DA, Favia M, Spera I, Campanella A, Lanza M, Castegna A. Metabolic Features of Brain Function with Relevance to Clinical Features of Alzheimer and Parkinson Diseases. Molecules. 2022 Jan 30;27(3):951. doi: 10.3390/molecules27030951. PMID: 35164216; PMCID: PMC8839962.
https://pubmed.ncbi.nlm.nih.gov/35164216/
77. Lilamand M, Mouton-Liger F, Di Valentin E, Sànchez Ortiz M, Paquet C. Efficacy and Safety of Ketone Supplementation or Ketogenic Diets for Alzheimer's Disease: A Mini Review. Front Nutr. 2022 Jan 17;8:807970. doi: 10.3389/fnut.2021.807970. PMID: 35111799; PMCID: PMC8803132.
https://pubmed.ncbi.nlm.nih.gov/35111799/
77a. Hersant H, Grossberg G. The Ketogenic Diet and Alzheimer's Disease. J Nutr Health Aging. 2022;26(6):606-614. doi: 10.1007/s12603-022-1807-7. PMID: 35718870.
https://pubmed.ncbi.nlm.nih.gov/35718870/
77b. Grammatikopoulou MG, Tousinas G, Balodimou C, Anastasilakis DA, Gkiouras K, Dardiotis E, Evangeliou AE, Bogdanos DP, Goulis DG. Ketogenic therapy for Parkinson's disease: A systematic review and synthesis without meta-analysis of animal and human trials. Maturitas. 2022 Jun 9;163:46-61. doi: 10.1016/j.maturitas.2022.06.001. Epub ahead of print. PMID: 35714419.
https://pubmed.ncbi.nlm.nih.gov/35714419/
Alzheimer, autophagy. Type 3 diabetes, transition metals
77c. Kishore, U. "Neurodegerative disease (ed.) In: Role of protein aggregation in neurodegenerative diseases." Tutar Y, Ozgur A, Tutar L (2013): 3.
https://www.intechopen.com/chapters/44540
77d. Willson J. Mitosis flips the switch on autophagy control. Nat Rev Mol Cell Biol. 2020 Jan;21(1):4-5. doi: 10.1038/s41580-019-0196-1. PMID: 31758162.
https://pubmed.ncbi.nlm.nih.gov/31758162/
77e. Almacellas E, Mauvezin C. Emerging roles of mitotic autophagy. J Cell Sci. 2022 Jun 1;135(11):jcs255802. doi: 10.1242/jcs.255802. Epub 2022 Jun 10. PMID: 35686549.
https://pubmed.ncbi.nlm.nih.gov/35686549/
77f. Del Barrio, Melisa, et al. "Metal-binding to amyloid-β peptide: coordination, aggregation, and reactive oxygen species production." Biometals in Neurodegenerative Diseases. Academic Press, 2017. 265-281.
https://www.sciencedirect.com/science/article/pii/B9780128045626000142
77g. Mocanu CS, Jureschi M, Drochioiu G. Aluminium Binding to Modified Amyloid-β Peptides: Implications for Alzheimer's Disease. Molecules. 2020 Oct 3;25(19):4536. doi: 10.3390/molecules25194536. PMID: 33022965; PMCID: PMC7582331.
https://pubmed.ncbi.nlm.nih.gov/33022965/
77h. Mold MJ, O'Farrell A, Morris B, Exley C. Aluminum and Tau in Neurofibrillary Tangles in Familial Alzheimer's Disease. J Alzheimers Dis Rep. 2021 Apr 9;5(1):283-294. doi: 10.3233/ADR-210011. PMID: 34113785; PMCID: PMC8150251.
https://pubmed.ncbi.nlm.nih.gov/34113785/
Alzheimer diabetic brain, type 3 diabetes, similarity with cancer
77i. Steen E, Terry BM, Rivera EJ, Cannon JL, Neely TR, Tavares R, Xu XJ, Wands JR, de la Monte SM. Impaired insulin and insulin-like growth factor expression and signaling mechanisms in Alzheimer's disease--is this type 3 diabetes? J Alzheimers Dis. 2005 Feb;7(1):63-80. doi: 10.3233/jad-2005-7107. PMID: 15750215.
https://pubmed.ncbi.nlm.nih.gov/15750215/
77j. Bartl J, Monoranu CM, Wagner AK, Kolter J, Riederer P, Grünblatt E. Alzheimer's disease and type 2 diabetes: two diseases, one common link? World J Biol Psychiatry. 2013 Apr;14(3):233-40. doi: 10.3109/15622975.2011.650204. Epub 2012 Feb 14. PMID: 22332892.
https://pubmed.ncbi.nlm.nih.gov/22332892/
77j. Nudelman KNH, McDonald BC, Lahiri DK, Saykin AJ. Biological Hallmarks of Cancer in Alzheimer's Disease. Mol Neurobiol. 2019 Oct;56(10):7173-7187. doi: 10.1007/s12035-019-1591-5. Epub 2019 Apr 16. PMID: 30993533; PMCID: PMC6728183.
https://pubmed.ncbi.nlm.nih.gov/30993533/
Parkinson/Alzheimer, Warburg effect, cell cycle
78. Atlante A, de Bari L, Bobba A, Amadoro G. A disease with a sweet tooth: exploring the Warburg effect in Alzheimer's disease. Biogerontology. 2017 Jun;18(3):301-319. doi: 10.1007/s10522-017-9692-x. Epub 2017 Mar 17. PMID: 28314935.
https://pubmed.ncbi.nlm.nih.gov/28314935/
79. Newington JT, Pitts A, Chien A, Arseneault R, Schubert D, Cumming RC. Amyloid beta resistance in nerve cell lines is mediated by the Warburg effect. PLoS One. 2011 Apr 26;6(4):e19191. doi: 10.1371/journal.pone.0019191. PMID: 21541279; PMCID: PMC3082554.
https://pubmed.ncbi.nlm.nih.gov/21541279/
80. Sonntag KC, Ryu WI, Amirault KM, Healy RA, Siegel AJ, McPhie DL, Forester B, Cohen BM. Late-onset Alzheimer's disease is associated with inherent changes in bioenergetics profiles. Sci Rep. 2017 Oct 25;7(1):14038. doi: 10.1038/s41598-017-14420-x. PMID: 29070876; PMCID: PMC5656579.
https://pubmed.ncbi.nlm.nih.gov/29070876/
81. Yang SQ, Tian Q, Li D, He SQ, Hu M, Liu SY, Zou W, Chen YJ, Zhang P, Tang XQ. Leptin mediates protection of hydrogen sulfide against 6-hydroxydopamine-induced Parkinson's disease: Involving enhancement in Warburg effect. Neurochem Int. 2020 May;135:104692. doi: 10.1016/j.neuint.2020.104692. Epub 2020 Feb 4. PMID: 32032636.
https://pubmed.ncbi.nlm.nih.gov/32032636/
81a. Moh C, Kubiak JZ, Bajic VP, Zhu X, Smith MA, Lee HG. Cell cycle deregulation in the neurons of Alzheimer's disease. Results Probl Cell Differ. 2011;53:565-76. doi: 10.1007/978-3-642-19065-0_23. PMID: 21630160; PMCID: PMC5925746.
https://pubmed.ncbi.nlm.nih.gov/21630160/
81b. Zekanowski C, Wojda U. Aneuploidy, chromosomal missegregation, and cell cycle reentry in Alzheimer's disease. Acta Neurobiol Exp (Wars). 2009;69(2):232-53. PMID: 19593337.
https://pubmed.ncbi.nlm.nih.gov/19593337/
81c. Barrio-Alonso E, Hernández-Vivanco A, Walton CC, Perea G, Frade JM. Cell cycle reentry triggers hyperploidization and synaptic dysfunction followed by delayed cell death in differentiated cortical neurons. Sci Rep. 2018 Sep 25;8(1):14316. doi: 10.1038/s41598-018-32708-4. PMID: 30254284; PMCID: PMC6156334.
https://pubmed.ncbi.nlm.nih.gov/30254284/
81d. Garcia-Ratés S, Greenfield S. When a trophic process turns toxic: Alzheimer's disease as an aberrant recapitulation of a developmental mechanism. Int J Biochem Cell Biol. 2022 Jun 30;149:106260. doi: 10.1016/j.biocel.2022.106260. Epub ahead of print. PMID: 35781081.
https://pubmed.ncbi.nlm.nih.gov/35781081/
81e. Wong GC, Chow KH. DNA Damage Response-Associated Cell Cycle Re-Entry and Neuronal Senescence in Brain Aging and Alzheimer's Disease. J Alzheimers Dis. 2022 Jul 11. doi: 10.3233/JAD-220203. Epub ahead of print. PMID: 35848025.
https://pubmed.ncbi.nlm.nih.gov/35848025/
Non-cancer diseases related to Warburg effect
7. Abdel-Haleem AM, Lewis NE, Jamshidi N, Mineta K, Gao X, Gojobori T. The Emerging Facets of Non-Cancerous Warburg Effect. Front Endocrinol (Lausanne). 2017 Oct 23;8:279. doi: 10.3389/fendo.2017.00279. PMID: 29109698; PMCID: PMC5660072.
https://pubmed.ncbi.nlm.nih.gov/29109698/
16. Chen Z, Liu M, Li L, Chen L. Involvement of the Warburg effect in non-tumor diseases processes. J Cell Physiol. 2018 Apr;233(4):2839-2849. doi: 10.1002/jcp.25998. Epub 2017 Jun 12. PMID: 28488732.
https://pubmed.ncbi.nlm.nih.gov/28488732/
17. Schwartz L, Henry M, Alfarouk KO, Reshkin SJ, Radman M. Metabolic Shifts as the Hallmark of Most Common Diseases: The Quest for the Underlying Unity. Int J Mol Sci. 2021 Apr 12;22(8):3972. doi: 10.3390/ijms22083972. PMID: 33921428; PMCID: PMC8068795.
https://pubmed.ncbi.nlm.nih.gov/33921428/
58. Zhu H, Bi D, Zhang Y, Kong C, Du J, Wu X, Wei Q, Qin H. Ketogenic diet for human diseases: the underlying mechanisms and potential for clinical implementations. Signal Transduct Target Ther. 2022 Jan 17;7(1):11. doi: 10.1038/s41392-021-00831-
Immune system
82. Hirschberger S et al. Ketone Bodies Improve Human CD8+ Cytotoxic T-Cell Immune Response During COVID-19 Infection. Front. Med., 16 June 2022
https://doi.org/10.3389/fmed.2022.923502
83. Hirschberger S, Strauß G, Effinger D, Marstaller X, Ferstl A, Müller MB, Wu T, Hübner M, Rahmel T, Mascolo H, Exner N, Heß J, Kreth FW, Unger K, Kreth S. Very-low-carbohydrate diet enhances human T-cell immunity through immunometabolic reprogramming. EMBO Mol Med. 2021 Aug 9;13(8):e14323. doi: 10.15252/emmm.202114323. Epub 2021 Jun 21. PMID: 34151532; PMCID: PMC8350890.
https://pubmed.ncbi.nlm.nih.gov/34151532/
84. Guo Y, Xie YQ, Gao M, Zhao Y, Franco F, Wenes M, Siddiqui I, Bevilacqua A, Wang H, Yang H, Feng B, Xie X, Sabatel CM, Tschumi B, Chaiboonchoe A, Wang Y, Li W, Xiao W, Held W, Romero P, Ho PC, Tang L. Metabolic reprogramming of terminally exhausted CD8+ T cells by IL-10 enhances anti-tumor immunity. Nat Immunol. 2021 Jun;22(6):746-756. doi: 10.1038/s41590-021-00940-2. Epub 2021 May 24. PMID: 34031618; PMCID: PMC7610876.
https://pubmed.ncbi.nlm.nih.gov/34031618/
85. Leone RD, Powell JD. Fueling the Revolution: Targeting Metabolism to Enhance Immunotherapy. Cancer Immunol Res. 2021 Mar;9(3):255-260. doi: 10.1158/2326-6066.CIR-20-0791. PMID: 33648947; PMCID: PMC8240594.
https://pubmed.ncbi.nlm.nih.gov/33648947/
86. Collins N, Belkaid Y. Control of immunity via nutritional interventions. Immunity. 2022 Feb 8;55(2):210-223. doi: 10.1016/j.immuni.2022.01.004. PMID: 35139351.
https://pubmed.ncbi.nlm.nih.gov/35139351/
Viruses, reprogram to Warburg effect
87. Darekar S, Georgiou K, Yurchenko M, Yenamandra SP, Chachami G, Simos G, Klein G, Kashuba E. Epstein-Barr virus immortalization of human B-cells leads to stabilization of hypoxia-induced factor 1 alpha, congruent with the Warburg effect. PLoS One. 2012;7(7):e42072. doi: 10.1371/journal.pone.0042072. Epub 2012 Jul 27. PMID: 22848707; PMCID: PMC3407085.
https://pubmed.ncbi.nlm.nih.gov/22848707/
88. Eisenreich W, Rudel T, Heesemann J, Goebel W. How Viral and Intracellular Bacterial Pathogens Reprogram the Metabolism of Host Cells to Allow Their Intracellular Replication. Front Cell Infect Microbiol. 2019 Mar 4;9:42. doi: 10.3389/fcimb.2019.00042. PMID: 30886834; PMCID: PMC6409310.
https://pubmed.ncbi.nlm.nih.gov/30886834/
88a. Stubbs BJ, Koutnik AP, Goldberg EL, Upadhyay V, Turnbaugh PJ, Verdin E, Newman JC. Investigating Ketone Bodies as Immunometabolic Countermeasures against Respiratory Viral Infections. Med (N Y). 2020 Dec 18;1(1):43-65. doi: 10.1016/j.medj.2020.06.008. Epub 2020 Jul 15. PMID: 32838361; PMCID: PMC7362813.
https://pubmed.ncbi.nlm.nih.gov/32838361/
88b. Allen CNS, Arjona SP, Santerre M, Sawaya BE. Hallmarks of Metabolic Reprogramming and Their Role in Viral Pathogenesis. Viruses. 2022 Mar 14;14(3):602. doi: 10.3390/v14030602. PMID: 35337009; PMCID: PMC8955778.
https://pubmed.ncbi.nlm.nih.gov/35337009/
88c. Icard P, Lincet H, Wu Z, Coquerel A, Forgez P, Alifano M, Fournel L. The key role of Warburg effect in SARS-CoV-2 replication and associated inflammatory response. Biochimie. 2021 Jan;180:169-177. doi: 10.1016/j.biochi.2020.11.010. Epub 2020 Nov 12. PMID: 33189832; PMCID: PMC7659517.
https://pubmed.ncbi.nlm.nih.gov/33189832/
88d. Ajaz S, McPhail MJ, Singh KK, Mujib S, Trovato FM, Napoli S, Agarwal K. Mitochondrial metabolic manipulation by SARS-CoV-2 in peripheral blood mononuclear cells of patients with COVID-19. Am J Physiol Cell Physiol. 2021 Jan 1;320(1):C57-C65. doi: 10.1152/ajpcell.00426.2020. Epub 2020 Nov 5. PMID: 33151090; PMCID: PMC7816428.
https://pubmed.ncbi.nlm.nih.gov/33151090/
88e. Kerstholt M, Netea MG, Joosten LAB. Borrelia burgdorferi hijacks cellular metabolism of immune cells: Consequences for host defense. Ticks Tick Borne Dis. 2020 May;11(3):101386. doi: 10.1016/j.ttbdis.2020.101386. Epub 2020 Feb 3. PMID: 32035898.
https://pubmed.ncbi.nlm.nih.gov/32035898/
Chronic fatigue, viruses, Warburg
89. Ruiz-Pablos M, Paiva B, Montero-Mateo R, Garcia N, Zabaleta A. Epstein-Barr Virus and the Origin of Myalgic Encephalomyelitis or Chronic Fatigue Syndrome. Front Immunol. 2021 Nov 15;12:656797. doi: 10.3389/fimmu.2021.656797. PMID: 34867935; PMCID: PMC8634673.
https://pubmed.ncbi.nlm.nih.gov/34867935/
90. Komaroff AL, Cho TA. Role of infection and neurologic dysfunction in chronic fatigue syndrome. Semin Neurol. 2011 Jul;31(3):325-37. doi: 10.1055/s-0031-1287654. Epub 2011 Sep 30. PMID: 21964849.
https://pubmed.ncbi.nlm.nih.gov/21964849/
90a. Fluge Ø, Mella O, Bruland O, Risa K, Dyrstad SE, Alme K, Rekeland IG, Sapkota D, Røsland GV, Fosså A, Ktoridou-Valen I, Lunde S, Sørland K, Lien K, Herder I, Thürmer H, Gotaas ME, Baranowska KA, Bohnen LM, Schäfer C, McCann A, Sommerfelt K, Helgeland L, Ueland PM, Dahl O, Tronstad KJ. Metabolic profiling indicates impaired pyruvate dehydrogenase function in myalgic encephalopathy/chronic fatigue syndrome. JCI Insight. 2016 Dec 22;1(21):e89376. doi: 10.1172/jci.insight.89376. PMID: 28018972; PMCID: PMC5161229.
https://pubmed.ncbi.nlm.nih.gov/28018972/
90b. Naviaux RK, Naviaux JC, Li K, Bright AT, Alaynick WA, Wang L, Baxter A, Nathan N, Anderson W, Gordon E. Metabolic features of chronic fatigue syndrome. Proc Natl Acad Sci U S A. 2016 Sep 13;113(37):E5472-80. doi: 10.1073/pnas.1607571113. Epub 2016 Aug 29. Erratum in: Proc Natl Acad Sci U S A. 2017 May 2;114(18):E3749. PMID: 27573827; PMCID: PMC5027464.
https://pubmed.ncbi.nlm.nih.gov/27573827/
Long COVID/Epstein Barr Virus
91. Su Y, Yuan D, Chen DG, Ng RH, Wang K, Choi J, Li S, Hong S, Zhang R, Xie J, Kornilov SA, Scherler K, Pavlovitch-Bedzyk AJ, Dong S, Lausted C, Lee I, Fallen S, Dai CL, Baloni P, Smith B, Duvvuri VR, Anderson KG, Li J, Yang F, Duncombe CJ, McCulloch DJ, Rostomily C, Troisch P, Zhou J, Mackay S, DeGottardi Q, May DH, Taniguchi R, Gittelman RM, Klinger M, Snyder TM, Roper R, Wojciechowska G, Murray K, Edmark R, Evans S, Jones L, Zhou Y, Rowen L, Liu R, Chour W, Algren HA, Berrington WR, Wallick JA, Cochran RA, Micikas ME; ISB-Swedish COVID-19 Biobanking Unit, Wrin T, Petropoulos CJ, Cole HR, Fischer TD, Wei W, Hoon DSB, Price ND, Subramanian N, Hill JA, Hadlock J, Magis AT, Ribas A, Lanier LL, Boyd SD, Bluestone JA, Chu H, Hood L, Gottardo R, Greenberg PD, Davis MM, Goldman JD, Heath JR. Multiple early factors anticipate post-acute COVID-19 sequelae. Cell. 2022 Mar 3;185(5):881-895.e20. doi: 10.1016/j.cell.2022.01.014. Epub 2022 Jan 25. PMID: 35216672; PMCID: PMC8786632.
https://pubmed.ncbi.nlm.nih.gov/35216672/
Ketones in oncology
92. Allen BG, Bhatia SK, Anderson CM, Eichenberger-Gilmore JM, Sibenaller ZA, Mapuskar KA, Schoenfeld JD, Buatti JM, Spitz DR, Fath MA. Ketogenic diets as an adjuvant cancer therapy: History and potential mechanism. Redox Biol. 2014;2:963-70. doi: 10.1016/j.redox.2014.08.002. Epub 2014 Aug 7. PMID: 25460731; PMCID: PMC4215472.
https://pubmed.ncbi.nlm.nih.gov/25460731/
93. Bozzetti F, Zupec-Kania B. Toward a cancer-specific diet. Clin Nutr. 2016 Oct;35(5):1188-95. doi: 10.1016/j.clnu.2015.01.013. Epub 2015 Jan 23. PMID: 25707910.
https://pubmed.ncbi.nlm.nih.gov/25707910/
94. Winter SF, Loebel F, Dietrich J. Role of ketogenic metabolic therapy in malignant glioma: A systematic review. Crit Rev Oncol Hematol. 2017 Apr;112:41-58. doi: 10.1016/j.critrevonc.2017.02.016. Epub 2017 Feb 20. PMID: 28325264.
https://pubmed.ncbi.nlm.nih.gov/28325264/
95. Weber DD, Aminzadeh-Gohari S, Tulipan J, Catalano L, Feichtinger RG, Kofler B. Ketogenic diet in the treatment of cancer - Where do we stand? Mol Metab. 2020 Mar;33:102-121. doi: 10.1016/j.molmet.2019.06.026. Epub 2019 Jul 27. PMID: 31399389; PMCID: PMC7056920.
https://pubmed.ncbi.nlm.nih.gov/31399389/
96. Poff A, Koutnik AP, Egan KM, Sahebjam S, D'Agostino D, Kumar NB. Targeting the Warburg effect for cancer treatment: Ketogenic diets for management of glioma. Semin Cancer Biol. 2019 Jun;56:135-148. doi: 10.1016/j.semcancer.2017.12.011. Epub 2017 Dec 30. PMID: 29294371; PMCID: PMC6927557.
https://pubmed.ncbi.nlm.nih.gov/29294371/
97. Hagihara K, Kajimoto K, Osaga S, Nagai N, Shimosegawa E, Nakata H, Saito H, Nakano M, Takeuchi M, Kanki H, Kagitani-Shimono K, Kijima T. Promising Effect of a New Ketogenic Diet Regimen in Patients with Advanced Cancer. Nutrients. 2020 May 19;12(5):1473. doi: 10.3390/nu12051473. PMID: 32438645; PMCID: PMC7284721.
https://pubmed.ncbi.nlm.nih.gov/32438645/
98. Li J, Zhang H, Dai Z. Cancer Treatment With the Ketogenic Diet: A Systematic Review and Meta-analysis of Animal Studies. Front Nutr. 2021 Jun 9;8:594408. doi: 10.3389/fnut.2021.594408. PMID: 34179051; PMCID: PMC8219874.
https://pubmed.ncbi.nlm.nih.gov/34179051/
99. Talib WH, Mahmod AI, Kamal A, Rashid HM, Alashqar AMD, Khater S, Jamal D, Waly M. Ketogenic Diet in Cancer Prevention and Therapy: Molecular Targets and Therapeutic Opportunities. Curr Issues Mol Biol. 2021 Jul 3;43(2):558-589. doi: 10.3390/cimb43020042. PMID: 34287243; PMCID: PMC8928964.
https://pubmed.ncbi.nlm.nih.gov/34287243/
100. Lan Y, Jin C, Kumar P, Yu X, Lenahan C, Sheng J. Ketogenic Diets and Hepatocellular Carcinoma. Front Oncol. 2022 May 4;12:879205. doi: 10.3389/fonc.2022.879205. PMID: 35600387; PMCID: PMC9115558.
https://pubmed.ncbi.nlm.nih.gov/35600387/
101. Dmitrieva-Posocco O, Wong AC, Lundgren P, Golos AM, Descamps HC, Dohnalová L, Cramer Z, Tian Y, Yueh B, Eskiocak O, Egervari G, Lan Y, Liu J, Fan J, Kim J, Madhu B, Schneider KM, Khoziainova S, Andreeva N, Wang Q, Li N, Furth EE, Bailis W, Kelsen JR, Hamilton KE, Kaestner KH, Berger SL, Epstein JA, Jain R, Li M, Beyaz S, Lengner CJ, Katona BW, Grivennikov SI, Thaiss CA, Levy M. β-Hydroxybutyrate suppresses colorectal cancer. Nature. 2022 May;605(7908):160-165. doi: 10.1038/s41586-022-04649-6. Epub 2022 Apr 27. PMID: 35477756.
https://pubmed.ncbi.nlm.nih.gov/35477756/
102. Yang L, TeSlaa T, Ng S, Nofal M, Wang L, Lan T, Zeng X, Cowan A, McBride M, Lu W, Davidson S, Liang G, Oh TG, Downes M, Evans R, Von Hoff D, Guo JY, Han H, Rabinowitz JD. Ketogenic diet and chemotherapy combine to disrupt pancreatic cancer metabolism and growth. Med (N Y). 2022 Feb 11;3(2):119-136. doi: 10.1016/j.medj.2021.12.008. PMID: 35425930; PMCID: PMC9004683.
https://pubmed.ncbi.nlm.nih.gov/35425930/
103. Barrea L, Caprio M, Tuccinardi D, Moriconi E, Di Renzo L, Muscogiuri G, Colao A, Savastano S; Obesity Programs of nutrition, Education, Research and Assessment (OPERA) group. Could ketogenic diet "starve" cancer? Emerging evidence. Crit Rev Food Sci Nutr. 2022;62(7):1800-1821. doi: 10.1080/10408398.2020.1847030. Epub 2020 Dec 4. PMID: 33274644.
https://pubmed.ncbi.nlm.nih.gov/33274644/
104. de Groot S, Lugtenberg RT, Cohen D, Welters MJP, Ehsan I, Vreeswijk MPG, Smit VTHBM, de Graaf H, Heijns JB, Portielje JEA, van de Wouw AJ, Imholz ALT, Kessels LW, Vrijaldenhoven S, Baars A, Kranenbarg EM, Carpentier MD, Putter H, van der Hoeven JJM, Nortier JWR, Longo VD, Pijl H, Kroep JR; Dutch Breast Cancer Research Group (BOOG). Fasting mimicking diet as an adjunct to neoadjuvant chemotherapy for breast cancer in the multicentre randomized phase 2 DIRECT trial. Nat Commun. 2020 Jun 23;11(1):3083. doi: 10.1038/s41467-020-16138-3. PMID: 32576828; PMCID: PMC7311547.
https://pubmed.ncbi.nlm.nih.gov/32576828/
105. Tran Q, Lee H, Kim C, Kong G, Gong N, Kwon SH, Park J, Kim SH, Park J. Revisiting the Warburg Effect: Diet-Based Strategies for Cancer Prevention. Biomed Res Int. 2020 Aug 4;2020:8105735. doi: 10.1155/2020/8105735. PMID: 32802877; PMCID: PMC7426758.
https://pubmed.ncbi.nlm.nih.gov/32802877/
SGLT2 inhibition in cancer
106. Scafoglio C, Hirayama BA, Kepe V, Liu J, Ghezzi C, Satyamurthy N, Moatamed NA, Huang J, Koepsell H, Barrio JR, Wright EM. Functional expression of sodium-glucose transporters in cancer. Proc Natl Acad Sci U S A. 2015 Jul 28;112(30):E4111-9. doi: 10.1073/pnas.1511698112. Epub 2015 Jul 13. PMID: 26170283; PMCID: PMC4522748.
https://pubmed.ncbi.nlm.nih.gov/26170283/
107. Nakano D, Kawaguchi T, Iwamoto H, Hayakawa M, Koga H, Torimura T. Effects of canagliflozin on growth and metabolic reprograming in hepatocellular carcinoma cells: Multi-omics analysis of metabolomics and absolute quantification proteomics (iMPAQT). PLoS One. 2020 Apr 28;15(4):e0232283. doi: 10.1371/journal.pone.0232283. PMID: 32343721; PMCID: PMC7188283.
https://pubmed.ncbi.nlm.nih.gov/32343721/
108. Wang Y, Yang L, Mao L, Zhang L, Zhu Y, Xu Y, Cheng Y, Sun R, Zhang Y, Ke J, Zhao D. SGLT2 inhibition restrains thyroid cancer growth via G1/S phase transition arrest and apoptosis mediated by DNA damage response signaling pathways. Cancer Cell Int. 2022 Feb 11;22(1):74. doi: 10.1186/s12935-022-02496-z. PMID: 35148777; PMCID: PMC8840070.
https://pubmed.ncbi.nlm.nih.gov/35148777/
108a. Sukkar SG, Muscaritoli M. A Clinical Perspective of Low Carbohydrate Ketogenic Diets: A Narrative Review. Front Nutr. 2021 Jul 12;8:642628. doi: 10.3389/fnut.2021.642628. PMID: 34322508; PMCID: PMC8310928.
https://pubmed.ncbi.nlm.nih.gov/34322508/
108b. Talib WH, Mahmod AI, Kamal A, Rashid HM, Alashqar AMD, Khater S, Jamal D, Waly M. Ketogenic Diet in Cancer Prevention and Therapy: Molecular Targets and Therapeutic Opportunities. Curr Issues Mol Biol. 2021 Jul 3;43(2):558-589. doi: 10.3390/cimb43020042. PMID: 34287243; PMCID: PMC8928964.
https://pubmed.ncbi.nlm.nih.gov/34287243/
108c. Barrea L, Caprio M, Tuccinardi D, Moriconi E, Di Renzo L, Muscogiuri G, Colao A, Savastano S; Obesity Programs of nutrition, Education, Research and Assessment (OPERA) group. Could ketogenic diet "starve" cancer? Emerging evidence. Crit Rev Food Sci Nutr. 2022;62(7):1800-1821. doi: 10.1080/10408398.2020.1847030. Epub 2020 Dec 4. PMID: 33274644.
https://pubmed.ncbi.nlm.nih.gov/33274644/
108d. Zhu H, Bi D, Zhang Y, Kong C, Du J, Wu X, Wei Q, Qin H. Ketogenic diet for human diseases: the underlying mechanisms and potential for clinical implementations. Signal Transduct Target Ther. 2022 Jan 17;7(1):11. doi: 10.1038/s41392-021-00831-w. PMID: 35034957; PMCID: PMC8761750.
https://pubmed.ncbi.nlm.nih.gov/35034957/
Hyperglycemia and diabetic kidney and heart failure
109. Sun HJ, Wu ZY, Cao L, Zhu MY, Liu TT, Guo L, Lin Y, Nie XW, Bian JS. Hydrogen Sulfide: Recent Progression and Perspectives for the Treatment of Diabetic Nephropathy. Molecules. 2019 Aug 6;24(15):2857. doi: 10.3390/molecules24152857. PMID: 31390847; PMCID: PMC6696501.
https://pubmed.ncbi.nlm.nih.gov/31390847/
110. Jung CY, Yoo TH. Pathophysiologic Mechanisms and Potential Biomarkers in Diabetic Kidney Disease. Diabetes Metab J. 2022 Mar;46(2):181-197. doi: 10.4093/dmj.2021.0329. Epub 2022 Mar 24. PMID: 35385633; PMCID: PMC8987689.
https://pubmed.ncbi.nlm.nih.gov/35385633/
110a. Shen S, Ji C, Wei K. Cellular Senescence and Regulated Cell Death of Tubular Epithelial Cells in Diabetic Kidney Disease. Front Endocrinol (Lausanne). 2022 Jun 28;13:924299. doi: 10.3389/fendo.2022.924299. PMID: 35837297; PMCID: PMC9273736.
https://pubmed.ncbi.nlm.nih.gov/35837297/
111. Jia G, Whaley-Connell A, Sowers JR. Diabetic cardiomyopathy: a hyperglycaemia- and insulin-resistance-induced heart disease. Diabetologia. 2018 Jan;61(1):21-28. doi: 10.1007/s00125-017-4390-4. Epub 2017 Aug 3. PMID: 28776083; PMCID: PMC5720913.
https://pubmed.ncbi.nlm.nih.gov/28776083/
112. Borghetti G, von Lewinski D, Eaton DM, Sourij H, Houser SR, Wallner M. Diabetic Cardiomyopathy: Current and Future Therapies. Beyond Glycemic Control. Front Physiol. 2018 Oct 30;9:1514. doi: 10.3389/fphys.2018.01514. PMID: 30425649; PMCID: PMC6218509.
https://pubmed.ncbi.nlm.nih.gov/30425649/
113. Nakamura K, Miyoshi T, Yoshida M, Akagi S, Saito Y, Ejiri K, Matsuo N, Ichikawa K, Iwasaki K, Naito T, Namba Y, Yoshida M, Sugiyama H, Ito H. Pathophysiology and Treatment of Diabetic Cardiomyopathy and Heart Failure in Patients with Diabetes Mellitus. Int J Mol Sci. 2022 Mar 25;23(7):3587. doi: 10.3390/ijms23073587. PMID: 35408946; PMCID: PMC8999085.
https://pubmed.ncbi.nlm.nih.gov/35408946/
114. Longo M, Scappaticcio L, Cirillo P, Maio A, Carotenuto R, Maiorino MI, Bellastella G, Esposito K. Glycemic Control and the Heart: The Tale of Diabetic Cardiomyopathy Continues. Biomolecules. 2022 Feb 8;12(2):272. doi: 10.3390/biom12020272. PMID: 35204778; PMCID: PMC8961546. https://pubmed.ncbi.nlm.nih.gov/35204778/
Evidence Based Medicine
114b. Sackett DL, Rosenberg WM, Gray JA, Haynes RB, Richardson WS. Evidence based medicine: what it is and what it isn't. BMJ. 1996 Jan 13;312(7023):71-2. doi: 10.1136/bmj.312.7023.71. PMID: 8555924; PMCID: PMC2349778.
https://pubmed.ncbi.nlm.nih.gov/8555924/
References Figure
115. Marchiq I, Pouysségur J. Hypoxia, cancer metabolism and the therapeutic benefit of targeting lactate/H(+) symporters. J Mol Med (Berl). 2016 Feb;94(2):155-71. doi: 10.1007/s00109-015-1307-x. Epub 2015 Jun 24. PMID: 26099350; PMCID: PMC4762928.
https://pubmed.ncbi.nlm.nih.gov/26099350/
116. Kroemer G, Pouyssegur J. Tumor cell metabolism: cancer's Achilles' heel. Cancer Cell. 2008 Jun;13(6):472-82. doi: 10.1016/j.ccr.2008.05.005. PMID: 18538731.
https://pubmed.ncbi.nlm.nih.gov/18538731/
117. Cardone RA, Alfarouk KO, Elliott RL, Alqahtani SS, Ahmed SBM, Aljarbou AN, Greco MR, Cannone S, Reshkin SJ. The Role of Sodium Hydrogen Exchanger 1 in Dysregulation of Proton Dynamics and Reprogramming of Cancer Metabolism as a Sequela. Int J Mol Sci. 2019 Jul 28;20(15):3694. doi: 10.3390/ijms20153694. PMID: 31357694; PMCID: PMC6696090.
https://pubmed.ncbi.nlm.nih.gov/31357694/
118. Birkeland ES, Koch LM, Dechant R. Another Consequence of the Warburg Effect? Metabolic Regulation of Na+/H+ Exchangers May Link Aerobic Glycolysis to Cell Growth. Front Oncol. 2020 Aug 18;10:1561. doi: 10.3389/fonc.2020.01561. PMID: 32974190; PMCID: PMC7462004.
https://pubmed.ncbi.nlm.nih.gov/32974190/
119. Shimoda LA, Fallon M, Pisarcik S, Wang J, Semenza GL. HIF-1 regulates hypoxic induction of NHE1 expression and alkalinization of intracellular pH in pulmonary arterial myocytes. Am J Physiol Lung Cell Mol Physiol. 2006 Nov;291(5):L941-9. doi: 10.1152/ajplung.00528.2005. Epub 2006 Jun 9. PMID: 16766575.
https://pubmed.ncbi.nlm.nih.gov/16766575/
120. Bender T, Martinou JC. The mitochondrial pyruvate carrier in health and disease: To carry or not to carry? Biochim Biophys Acta. 2016 Oct;1863(10):2436-42. doi: 10.1016/j.bbamcr.2016.01.017. Epub 2016 Jan 26. PMID: 26826034.
https://pubmed.ncbi.nlm.nih.gov/26826034/
121. Zangari J, Petrelli F, Maillot B, Martinou JC. The Multifaceted Pyruvate Metabolism: Role of the Mitochondrial Pyruvate Carrier. Biomolecules. 2020 Jul 17;10(7):1068. doi: 10.3390/biom10071068. PMID: 32708919; PMCID: PMC7407832.
https://pubmed.ncbi.nlm.nih.gov/32708919/
122. Ward PS, Thompson CB. Metabolic reprogramming: a cancer hallmark even warburg did not anticipate. Cancer Cell. 2012 Mar 20;21(3):297-308. doi: 10.1016/j.ccr.2012.02.014. PMID: 22439925; PMCID: PMC3311998.
https://pubmed.ncbi.nlm.nih.gov/22439925/
123. Snyder J, Zhai R, Lackey AI, Sato PY. Changes in Myocardial Metabolism Preceding Sudden Cardiac Death. Front Physiol. 2020 Jun 16;11:640. doi: 10.3389/fphys.2020.00640. PMID: 32612538; PMCID: PMC7308560.
https://pubmed.ncbi.nlm.nih.gov/32612538/
124. Yamamoto T, Sano M. Deranged Myocardial Fatty Acid Metabolism in Heart Failure. Int J Mol Sci. 2022 Jan 17;23(2):996. doi: 10.3390/ijms23020996. PMID: 35055179; PMCID: PMC8779056.
https://pubmed.ncbi.nlm.nih.gov/35055179/
125. Almacellas E, Mauvezin C. Emerging roles of mitotic autophagy. J Cell Sci. 2022 Jun 1;135(11):jcs255802. doi: 10.1242/jcs.255802. Epub 2022 Jun 10. PMID: 35686549.
https://pubmed.ncbi.nlm.nih.gov/35686549/
126. Schwartz L, Henry M, Alfarouk KO, Reshkin SJ, Radman M. Metabolic Shifts as the Hallmark of Most Common Diseases: The Quest for the Underlying Unity. Int J Mol Sci. 2021 Apr 12;22(8):3972. doi: 10.3390/ijms22083972. PMID: 33921428; PMCID: PMC8068795.
https://pubmed.ncbi.nlm.nih.gov/33921428/
Senescent cells
110a. Shen S, Ji C, Wei K. Cellular Senescence and Regulated Cell Death of Tubular Epithelial Cells in Diabetic Kidney Disease. Front Endocrinol (Lausanne). 2022 Jun 28;13:924299. doi: 10.3389/fendo.2022.924299. PMID: 35837297; PMCID: PMC9273736.
https://pubmed.ncbi.nlm.nih.gov/35837297/
127. van Deursen JM. The role of senescent cells in ageing. Nature. 2014 May 22;509(7501):439-46. doi: 10.1038/nature13193. PMID: 24848057; PMCID: PMC4214092.
https://pubmed.ncbi.nlm.nih.gov/24848057/
128. Lujambio A. To clear, or not to clear (senescent cells)? That is the question. Bioessays. 2016 Jul;38 Suppl 1:S56-64. doi: 10.1002/bies.201670910. PMID: 27417123.
https://pubmed.ncbi.nlm.nih.gov/27417123/
128a. Sabbatinelli J, Prattichizzo F, Olivieri F, Procopio AD, Rippo MR, Giuliani A. Where Metabolism Meets Senescence: Focus on Endothelial Cells. Front Physiol. 2019 Dec 18;10:1523. doi: 10.3389/fphys.2019.01523. PMID: 31920721; PMCID: PMC6930181.
https://pubmed.ncbi.nlm.nih.gov/31920721/
128b. Wiley CD, Campisi J. The metabolic roots of senescence: mechanisms and opportunities for intervention. Nat Metab. 2021 Oct;3(10):1290-1301. doi: 10.1038/s42255-021-00483-8. Epub 2021 Oct 18. PMID: 34663974; PMCID: PMC8889622.
https://pubmed.ncbi.nlm.nih.gov/34663974/
128c. Chapman J, Fielder E, Passos JF. Mitochondrial dysfunction and cell senescence: deciphering a complex relationship. FEBS Lett. 2019 Jul;593(13):1566-1579. doi: 10.1002/1873-3468.13498. Epub 2019 Jun 27. PMID: 31211858.
https://pubmed.ncbi.nlm.nih.gov/31211858/
129. Xu M, Pirtskhalava T, Farr JN, Weigand BM, Palmer AK, Weivoda MM, Inman CL, Ogrodnik MB, Hachfeld CM, Fraser DG, Onken JL, Johnson KO, Verzosa GC, Langhi LGP, Weigl M, Giorgadze N, LeBrasseur NK, Miller JD, Jurk D, Singh RJ, Allison DB, Ejima K, Hubbard GB, Ikeno Y, Cubro H, Garovic VD, Hou X, Weroha SJ, Robbins PD, Niedernhofer LJ, Khosla S, Tchkonia T, Kirkland JL. Senolytics improve physical function and increase lifespan in old age. Nat Med. 2018 Aug;24(8):1246-1256. doi: 10.1038/s41591-018-0092-9. Epub 2018 Jul 9. PMID: 29988130; PMCID: PMC6082705.
https://pubmed.ncbi.nlm.nih.gov/29988130/
130. Jarreau PB. Don’t Be a Zombie: Senolytics, Exercise and Fasting Fight Off Senescent Cells. Juli 25, 2018
https://medium.com/lifeomic/dont-be-a-zombie-senolytics-exercise-and-fas...
131. Lecot P, Alimirah F, Desprez PY, Campisi J, Wiley C. Context-dependent effects of cellular senescence in cancer development. Br J Cancer. 2016 May 24;114(11):1180-4. doi: 10.1038/bjc.2016.115. Epub 2016 May 3. PMID: 27140310; PMCID: PMC4891501.
https://pubmed.ncbi.nlm.nih.gov/27140310/
132. Wilkinson HN, Hardman MJ. Senescence in Wound Repair: Emerging Strategies to Target Chronic Healing Wounds. Front Cell Dev Biol. 2020 Aug 11;8:773. doi: 10.3389/fcell.2020.00773. PMID: 32850866; PMCID: PMC7431694.
https://pubmed.ncbi.nlm.nih.gov/32850866/\
133. Blagosklonny MV. Cell cycle arrest is not senescence. Aging (Albany NY). 2011 Feb;3(2):94-101. doi: 10.18632/aging.100281. PMID: 21297220; PMCID: PMC3082019.
https://pubmed.ncbi.nlm.nih.gov/21297220/
134. Coppé JP, Desprez PY, Krtolica A, Campisi J. The senescence-associated secretory phenotype: the dark side of tumor suppression. Annu Rev Pathol. 2010;5:99-118. doi: 10.1146/annurev-pathol-121808-102144. PMID: 20078217; PMCID: PMC4166495.
https://pubmed.ncbi.nlm.nih.gov/20078217/
135. Papazoglu C, Mills AA. p53: at the crossroad between cancer and ageing. J Pathol. 2007 Jan;211(2):124-33. doi: 10.1002/path.2086. PMID: 17200941.
https://pubmed.ncbi.nlm.nih.gov/17200941/
136. Frasca D, Saada YB, Garcia D, Friguet B. Effects of cellular senescence on metabolic pathways in non-immune and immune cells. Mech Ageing Dev. 2021 Mar;194:111428. doi: 10.1016/j.mad.2020.111428. Epub 2020 Dec 28. PMID: 33383073; PMCID: PMC7882031.
https://pubmed.ncbi.nlm.nih.gov/33383073/