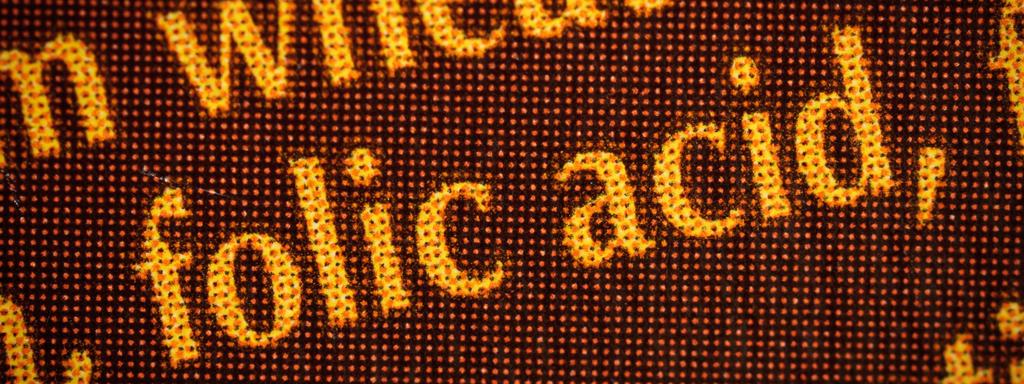
Foliumzuur is vooral bekend omwille van de relatie tussen deficiëntie en een verhoogd risico op neuraalbuis- en andere geboortedefecten. Een probleem dat ondanks decennialange sensibiliserings- en fortificatieprogramma's en suppletie nog lang de wereld niet uit is. En dat heel wat verder reikt dan spina bifida ...
Periconceptuele en prenatale voeding/folaatstatus beïnvloedt de methyleringspatronen en via deze epigenetische modulatie kan prenatale malnutritie een voor- of nadelig metabool fenotype induceren voor de rest van het leven.
Foliumzuursuppletie blijft ook postnataal belangrijk voor het kind, maar ook later, doorheen het hele leven. Er is de relatie met genstabiliteit en kanker, hart- en vaatziekten, leeftijdsgerelateerde fysische, psychische en cognitieve deficits.
Eigenlijk is er zoveel te zeggen over foliumzuur dat het bijna onbegonnen werk is om het in een artikel te gieten. Daarom vooral nadruk op wat nieuw en minder algemeen bekend is.
Beste bezoeker, u heeft geen toegang.
Enkel (web)abonnees hebben toegang tot tijdschriftartikelen. Het webabonnement is nog in de maak.
U kunt zich wel alvast (gratis) registreren en tal van andere webartikelen raadplegen!
Auteur
Verschenen in
Referenties
1. http://www.who.int/nutrition/events/2014_WHOCDC_symposium_folatepreventa...
2. Purnell BA. A legacy that transcends genes. Science 15 August 2014: 742-743.
3. Susiarjo M, Bartolomei MS. Perspective Epigenetics You are what you eat, but what about your DNA? Science 15 August 2014: 733-734.
4. Dominguez-Salas P et al. Maternal nutrition at conception modulates DNA methylation of human metastable epialleles. Nature 2014; 5: art;nr: 3746.
5. Nys M. De werking van foliumzuur blijkt steeds veelzijdiger. Deel 1. Orthofyto 2002; 38:69-70.
6. Nys M. De werking van foliumzuur blijkt steeds veelzijdiger. Deel 2. Orthofyto 2002; 39:105-106.
7. van Mil NH, Steegers-Theunissen RP et al. Determinants of maternal pregnancy one-carbon metabolism and newborn human DNA methylation profiles. Reproduction. 2014 Dec;148(6):581-92.
8. http://www.cliffsnotes.com/sciences/biology/biochemistry-ii/amino-acid-m...
9. Kalhan SC. One Carbon Metabolism, Fetal Growth and Long Term Consequences. Nestle Nutr Inst Workshop Ser. 2013; 74: 127–138.
10. Yajnik CS1, Deshmukh US. Fetal programming: maternal nutrition and role of one-carbon metabolism. Rev Endocr Metab Disord. 2012 Jun;13(2):121-7.
11. Kalhan SC, Marczewski SE. Methionine, homocysteine, one carbon metabolism and fetal growth. Rev Endocr Metab Disord. 2012 Jun;13(2):109-19.
12. Kurpad A. Discussion on one-carbon metabolism, fetal growth and long-term consequences. Nestle Nutr Inst Workshop Ser. 2013;74:139-44.
13. Steegers-Theunissen RP, Twigt J, Pestinger V, Sinclair KD. The periconceptional period, reproduction and long-term health of offspring: the importance of one-carbon metabolism. Hum Reprod Update. 2013 Nov-Dec;19(6):640-55.
14. Crider KS et al. Folate and DNA Methylation: A Review of Molecular Mechanisms and the Evidence for Folate’s Role. Adv Nutr. Jan 2012; 3(1): 21–38.
15. Stover P. Polymorphisms in 1-Carbon Metabolism, Epigenetics and Folate-Related Pathologies. J Nutrigenet Nutrigenomics. 2012 February; 4(5): 293–305.
16. Boyles AL et al. Folate and One-Carbon Metabolism Gene Polymorphisms and Their Associations With Oral Facial Clefts. Am J Med Genet A. 2008 15; 146(4): 440–449
17. Beaudin AE, Stover PJ. Folate-mediated one-carbon metabolism and neural tube defects: balancing genome synthesis and gene expression. Birth Defects Res C Embryo Today. 2007 Sep;81(3):183-203.
18. Suarez L1, Felkner M, Brender JD, Canfield M, Zhu H, Hendricks KA. Brownsville cluster. Birth Defects Res A Clin Mol Teratol. 2012 Nov;94(11):882-92.
19. Bortolus R et al. Prevention of congenital malformations and other adverse pregnancy outcomes with 4.0 mg of folic acid: community-based randomized clinical trial in Italy and the Netherlands. BMC Pregnancy Childbirth. 2014 May 13;14:166.
20. Barua et al. Folic acid supplementation in pregnancy and implications in health and disease. Journal of Biomedical Science 2014 21:77
21. Schroeder DI, LaSalle JM. How has the study of the human placenta aided our understanding of partially methylated genes? Epigenomics 2013; 5(6):645-54.
22. Barua et al. Folic acid supplementation in pregnancy and implications in health and disease. Journal of Biomedical Science 2014 21:77.
23. Cao Y et al. The association of idiopathic recurrent early pregnancy loss with polymorphisms in folic acid metabolism-related genes. Genes Nutr. 2014 May;9(3):402.
24. Wen SW et al. Folic acid supplementation in early second trimester and the risk of pre-eclampsia. Am J Obst Gyn 2008, 198(1) 45.e1-45.e7.
25. Wen SW et al. Effect of folic acid supplementation in pregnancy on preeclampsia: the folic acid clinical trial study. J Pregnancy2013; 294312.
26. D'Souza V et al. Counteracting oxidative stress in pregnancy through modulation of maternal micronutrients and omega-3 fatty acids. Curr Med Chem 2013; 20(37):4777-83.
27. Kemse NG, Kale AA, Joshi SR. A combined supplementation of omega-3 fatty acids and micronutrients (folic acid, vitamin B12) reduces oxidative stress markers in a rat model of pregnancy induced hypertension. PLoS One 2014 Nov 18;9(11):e111902.
28. Dean SV et al. Preconception care: nutritional risks and interventions. Reprod Health 2014 Sep 26;11 Suppl 3:S3.
29. Barbosa PR et al. Association between decreased vitamin levels and MTHFR, MTR and MTRR gene polymorphisms as determinants for elevated total homocysteine concentrations in pregnant women. Eur J Clin Nutr. 2008 Aug;62(8):1010-21.
30. Coppedè F et al. Folate gene polymorphisms and the risk of Down syndrome pregnancies in young Italian women. Am J Med Genet A. 2006 May 15;140(10):1083-91.
31. Field MS et al. Reduced MTHFD1 activity in male mice perturbs folate- and choline-dependent one-carbon metabolism as well as transsulfuration. J Nutr. 2013 Jan;143(1):41-5.
32. Gava MM, Barbosa CP et al. Polymorphisms in folate-related enzyme genes in idiopathic infertile Brazilian men. Reprod Sci 2011 Dec;18(12):1267-72.
33. Liao YP et al. Folate gene polymorphism and the risk of Down syndrome pregnancies in young Chinese women. Yi Chuan. 2010 May;32(5):461-6.
34. Hobbs CA et al. Polymorphisms in genes involved in folate metabolism as maternal risk factors for Down syndrome. Am J Hum Genet. 2000 Sep;67(3):623-30.
35. Rai V et al. Maternal methylenetetrahydrofolate reductase C677T polymorphism and down syndrome risk: a meta-analysis from 34 studies. PLoS One. 2014 Sep 29;9(9):e108552.
36. Schroeder DI, LaSalle JM. How has the study of the human placenta aided our understanding of partially methylated genes? Epigenomics 2013; 5(6):645-45.
Epigenetische printing (zie: imprinting)
37. Barlow DP, Bartolomei MS. Genomic Imprinting in Mammals. http://cshperspectives.cshlp.org/content/6/2/a018382.full
38. Barlow DP. 2011. Genomic imprinting: A mammalian epigenetic discovery model. Annu Rev Genet 45: 379–403.
39. Bartolomei MS, Ferguson-Smith AC. 2011. Mammalian genomic imprinting. Cold Spring Harb Perspect Biol doi:10.1101/cshperspect.a002592.
40. Barlow DP, Bartolomei MS. Epigenetics Genomic Imprinting in Mammals. Cold Spring Harb Perspect Biol 2014;6:a018382
41. Li E, Zhang Y. Epigenetics DNA Methylation in Mammals. Cold Spring Harb Perspect Biol doi: 10.1101/cshperspect.a019133
42. Fedoriw A, Mugford J, Magnuson T. Mammalian Development Genomic Imprinting and Epigenetic Control of Development. Cold Spring Harb Perspect Biol 2012;4:a008136
43. Bartolomei MS, Ferguson-Smith AC. Germ Cells Mammalian Genomic Imprinting. Cold Spring Harb Perspect Biol 2011;3:a002592
44. Murphy SK, Huang Z, Hoyo C. Differentially methylated Regions of Imprinted Genes in Prenatal, Perinatal and Postnatal Human Tissues. PLOS One Published: July 13, 2012. DOI: 10.1371/journal.pone.0040924SK,
45. Hackett JA, Surani MA. DNA methylation dynamics during the mammalian life cycle. Philos Trans R Soc Lond B Biol Sci. Jan 5, 2013; 368(1609): 20110328.
46. Grossniklaus U, Paro R. Epigenetics Transcriptional Silencing by Polycomb-Group Proteins. Cold Spring Harb Perspect Biol November 2014;6:a019331
47. Blewitt M, Whitelaw E. Epigenetics The Use of Mouse Models to Study Epigenetics. Cold Spring Harb Perspect Biol November 2013;5:a017939
48. Ensminger AW, Chess A. Coordinated replication timing of monoallelically expressed genes along human autosomes. Hum. Mol. Genet. 2004. 13:651–58
49. Li E, Beard C, Jaenisch R. Role for DNA methylation in genomic imprinting. Nature 1993. 366:362–65
50. Lewis BA, Hanover JA. O-GlcNAc and the Epigenetic Regulation of Gene Expression. J Biol Chem 2014 289 (50) 34440-34448
51. Eskes TK. Abnormal folate metabolism in mothers with Down syndrome offspring: review of the literature. Eur J Obstet Gynecol Reprod Biol. 2006 Feb 1;124(2):130-3
52. Liao YP et al. Folate gene polymorphism and the risk of Down syndrome pregnancies in young Chinese women. Yi Chuan. 2010 May;32(5):461-6.
53. Cassidy S, Dykens E, Williams C. Prader-Willi and Angelman syndromes: sister imprinted disorders. Am. J. Med. Genet. 2000. 97:136–46
54. Ingrosso D, Cimmino A, Perna AF, Masella L, De Santo NG, et al. Folate treatment and unbalanced methylation and changes of allelic expression induced by hyperhomocysteinaemia in patients with uraemia. Lancet 2003; 361:1693–99
55. Levine MA, Germain-Lee E, Jan dB. Genetic basis for resistance to parathyroid hormone. Horm. Res. 2003. 60(Suppl.) 3:87–95
56. Weksberg R, Smith AC, Squire J, Sadowski P. Beckwith-Wiedemann syndrome demonstrates a role for epigenetic control of normal development. Hum. Mol. Genet. 2003. 121:R61–68
57. Williams CA, Zori RT, Hendrickson J, Stalker H, Marum T, et al. Angelman syndrome. Curr. Probl. Pediatr. 1995. 25:216–31
58. Bussemaker HJ et al. Evolving insights on how cytosine methylation affects protein-DNA binding. Briefings in Functional Genomics 2014: elu040v1-elu040.
59. Murrell A. Genomic Imprinting and Cancer: From Primordial Germ Cells to Somatic Cells. TheScientificworldJournal 2006; 6:1888-1910.
60. Wilkins JF, Ubeda F. Diseases associated with genomic imprinting. Prog Mol Biol Transl Sci. 2011; 101:401-45.
61. Netchine I et al. Epigenetic anomalies in childhood growth disorders. Nestle Nutr Inst Workshop Ser. 2013;71:65-73.
62. Soejima H, Higashimoto K. Epigenetic and genetic alterations of the imprinting disorder Beckwith-Wiedemann syndrome and related disorders. J Hum Genet 2013 Jul;58(7):402-9.
63. Le Bouc Y et al. Epigenetics, genomic imprinting and developmental disorders. Bull Acad Natl Med 2010 Feb;194(2):287-97; discussion 297-300.
64. Uribe-Lewis S et al. Molecular mechanisms of genomic imprinting and clinical implications for cancer. Expert Rev Mol Med 2011 Jan 25;13:e2.
65. Joyce JA, Schofiled PN. Genomic imprinting and cancer. Mol Pathol. Aug 1998; 51(4): 185–190.
66. Lim DH, Maher ER. Genomic imprinting syndromes and cancer. Adv Genet 2010;70:145-75.
67. Amor DJ, Halliday J. A review of known imprinting syndromes and their association with assisted reproduction technologies. Hum Reprod 2008; 23(12):2826-34.
68. Owen CM, Segars JH Jr. Imprinting disorders and assisted reproductive technology. Semin Reprod Med 2009 Sep;27(5):417-28.
69. Padmanabhan N, Watson ED et al. Mutation in Folate Metabolism Causes Epigenetic Instability and Transgenerational Effects on Development. Cell, 2013; 155 (1): 81
70. University of Cambridge. Folic acid deficiency can affect the health of great, great grandchildren.. ScienceDaily, 26 Sep 2013. www.sciencedaily.com/releases/2013/09/130926123450.htm
71. Ranvourt RC, Harris HR, Barault L, Michels KB. The prevalence of loss of imprinting of H19 and IGF2 at birth. FASEB 2013; 27(8):3335-3343
72. Nelissen ECM, van Montfoort APA et al. Altered gene expression in human placentas after IVF/ICSI. Hum Reprod December 1, 2014 29:2821-2831
73. Radford EJ, Ferguson-Smith AC et al. In utero undernourishment perturbs the adult sperm methylome and intergenerational metabolism. Science 2014; 345 no. 6198
74. McRae AF et al. Contribution of genetic variation to transgenerational inheritance of DNA methylation. Genome Biology 2014, 15:R73.
75. Radford EJ, Ferguson-Smith AC et al. In utero undernourishment perturbs the adult sperm methylome and intergenerational metabolism. Science 2014; 345 no. 6198
76. Soubry A et al. Paternal obesity is associated with IGF2 hypomethylation in newborns: results from a Newborn Epigenetics Study (NEST) cohort. BMC Med. 2013 Feb 6;11:29.
77. Soubry A, Hoyo C et al. Newborns of obese parents have altered DNA methylation patterns at imprinted genes. Int J Obes (Lond). 2013 Oct 25. [Epub ahead of print]
78. Soubry A, Hoyo C, Jirtle RL, Murphy SK. A paternal environmental legacy: Evidence for epigenetic inheritance through the male germ line. Bioessays. Apr 2014; 36(4): 359–371.
79. Dattilo M et al. The importance of the one carbon cycle nutritional support in human male fertility: a preliminary clinical report. Reprod Biol Endocrinol. 2014; 12: 71.
80. Jiang YH, Bressler J, Beaudet AL. Epigenetics and Human Disease. AnnuRev Genomics Hum Genet 2004; 5:479-510.
81. Wei Y, Schatten H, Sun QY. Environmental epigenetic inheritance through gametes and implications for human reproduction. Hum Reprod Update 2014: dmu061v1-dmu061.
82. Ubeda F, Wilkins JF. Imprinted genes and human disease: an evolutionary perspective. Adv Exp Med Biol. 2008;626:101-15.
83. Benyshek DC. The "early life" origins of obesity-related health disorders: new discoveries regarding the intergenerational transmission of developmentally programmed traits in the global cardiometabolic health crisis. Am J Phys Anthropol. 2013 Dec;152 S57:79-93.
84. Turan N et al. DNA methylation differences at growth related genes correlate with birth weight: a molecular signature linked to developmental origins of adult disease? BMC Med Genomics. 2012 Apr 12;5:10.
85. Vickers MH. Developmental programming and transgenerational transmission of obesity. Hum Reprod Update. 2014 Jan-Feb;20(1):63-75.
86. Aiken CE, Ozanne SE. Transgenerational developmental programming. Ann Nutr Metab. 2014;64 S1:26-34.
87. Lane M, Robker RL, Robertson SA. Parenting from before conception. Science 2014: 756-760. -
88. Radford EJ, Ferguson-Smith AC et al. In utero undernourishment perturbs the adult sperm methylome and intergenerational metabolism. Science 2014; 345 no. 6198
89. Reprod Sci. 2011 Dec;18(12):1267-72. doi: 10.1177/1933719111411729. Epub 2011 Jul 20.
90. Tobi AW et al. DNA methylation signatures link prenatal famine exposure to growth and metabolism. Nature Communications 2014; 5, art.nr 5592.
91. Kaati G, Bygren LO, Edvinsson S. Cardiovascular and diabetes mortality determined by nutrition during parents’ and grandparents’ slow growth period. Eur. J. Hum. Genet. 2002. 10:682–88
92. Petronis A. Human morbid genetics revisited: relevance of epigenetics. Trends Genet. 2001. 17:142–46
93. Beaudet A, Zoghbi H. 2014. Epigenetics and human disease. Cold Spring Harb Perspect Biol doi:10.1101/cshperspect.a019497.
94. Jiang Y-H, Bressler J, Beaudet AL. Epigenetics and Human Disease. Annu Rev Genomics Hum Gent 2004; 5:479-510.
95. Gabory A, Attig L, Junien C. Developmental programming and epigenetics. Am J Clin Nutr. 2011 Dec;94(6 Suppl):1943S-1952S.
96. Saffery R. Epigenetic change as the major mediator of fetal programming in humans: are we there yet? Ann Nutr Metab. 2014;64(3-4):203-7.
97. Vaag A et al. Genetic, nongenetic and epigenetic risk determinants in developmental programming of type 2 diabetes.Acta Obstet Gynecol Scand. 2014 Nov;93(11):1099-108.
98. Lane RH. Fetal Programming, Epigenetics, and Adult Onset Disease. Clin Perinatol. 2014 Dec;41(4):815-831
99. Quilter CR et al. Impact on offspring methylation patterns of maternal gestational diabetes mellitus and intrauterine growth restraint suggest common genes and pathways linked to subsequent type 2 diabetes risk. FASEB J. 2014 Nov;28(11):4868-79.
100. Keverne EB. Significance of epigenetics for understanding brain development, brain evolution and behaviour. Neuroscience. 2014 Apr 4;264:207-17.
101. Tycko B, Morison IM. Physiological functions of imprinted genes. J. Cell Physiol 2002. 192:245–58
102. Petronis A. The genes for major psychosis: aberrant sequence or regulation? Neuropsychopharmacology 2000. 23:1–12
103. Debnath M, Venkatasubramanian G, Berk M. Fetal programming of schizophrenia: Select mechanisms. Neurosci Biobehav Rev. 2014 Dec 10;49C:90-104.
104. Petronis A. Epigenetics and bipolar disorder: new opportunities and challenges. Am. J. Med. Genet. 2003. 123C:65–75
105. Judson H, Hayward BE, Sheridan E, Bonthron DT. A global disorder of imprinting in the human female germ line. Nature 2002. 416:539–42
106. Kan PX, Popendikyte V, Kaminsky ZA, Yolken RH, Petronis A. Epigenetic studies of genomic retroelements in major psychosis. Schizophr. Res. 2004. 67:95–106
107. Lok A et al. The one-carbon-cycle and methylenetetrahydrofolate reductase (MTHFR) C677T polymorphism in recurrent major depressive disorder; influence of antidepressant use and depressive state?J Affect Disord. 2014 Sep;166:115-23.
108. Coşar A, Ipçioğlu OM, Ozcan O, Gültepe M. Folate and homocysteine metabolisms and their roles in the biochemical basis of neuropsychiatry. Turk J Med Sci. 2014;44(1):1-9.
109. Kim JM et al. Changes in folate, vitamin B12 and homocysteine associated with incident dementia. J Neurol Neurosurg & Psych Published online ahead of print, doi 10.1136/jnnp.2007.131482
110. Iskandar BJ, Hogan KJ et al. Folate regulation of axonal regeneration in the rodent central nervous system through DNA methylation. J Clin Invest, 2010; DOI: 10.1172/JCI40000
111. Kronenberg, Endres M. Neuronal injury: folate to the rescue? J Clin Invest 2010 May;120(5):1383-6.
112. Fenech M. Folate, DNA damage and the aging brain. Mech Ageing Dev. 2010 Apr;131(4):236-41.
113. Hui SP et al. Genome wide expression profiling during spinal cord regeneration identifies comprehensive cellular responses in zebrafish. PLoS One 2014 Jan 20;9(1):e84212
114. J Clin Invest. Understanding how folic acid might help heal brain and spinal cord injuries. http://www.sciencedaily.com/releases/2010/04/100426181708.htm
115. Kronenberg G, Colla M, Endres M. Folic acid, neurodegenerative and neuropsychiatric disease. Curr Mol Med. 2009 Apr;9(3):315-23.
116. Kalani A et al. Nutri-epigenetics Ameliorates Blood–Brain Barrier Damage and Neurodegeneration in Hyperhomocysteinemia: Role of Folic Acid. J Mol Neurosci. 2014 February;
117. Huo Y et al. Efficacy of folic acid supplementation in stroke prevention: new insight from a meta-analysis. Int J Clin Pract 2012 Jun;66(6):544-51.
118. Qin X et al. Homocysteine-lowering therapy with folic acid is effective in cardiovascular disease prevention in patients with kidney disease: a meta-analysis of randomized controlled trials. Clin Nutr 2013 Oct;32(5):722-7. doi: 10.1016/j.clnu.2012.12.009. Epub 2012 Dec 28.
119. Qin X et al. Folic acid supplementation with and without vitamin B6 and revascularization risk: a meta-analysis of randomized controlled trials. Clin Nutr 2014 Aug;33(4):603-12.
120. The Heart Outcomes Prevention Evaluation (HOPE) 2 Investigators. Homocysteine Lowering with Folic Acid and B Vitamins in Vascular Disease. N Engl J Med 2006; 354:1567-1577
121. Marosi K et al. The role of homocysteine and methylenetetrahydrofolate reductase, methionine synthase, methionine synthase reductase polymorphisms in the development of cardiovascular diseases and hypertension. Orv Hetil. 2012 Mar 25;153(12):445-53.
122. Joseph J, Loscalzo J. Methoxistasis: Integrating the Roles of Homocysteine and Folic Acid in Cardiovascular Pathobiology. Nutrients. 2013 August; 5(8): 3235–3256.
123. Liew S, Gupta ED. Methylenetetrahydrofolate reductase (MTHFR) C677T polymorphism: Epidemiology, metabolism and the associated diseases. Eur J Med Genet2015 Jan;58(1):1-10.
124. Khandanpour N, Jennings BA et al. Peripheral arterial disease and methylenetetrahydrofolate reductase (MTHFR) C677T mutations: A case-control study and meta-analysis. J Vasc Surg. 2009 Mar;49(3):711-8.
125. Bhargava S, Ali A, Parakh R, Saxena R, Srivastava LM. Higher incidence of C677T polymorphism of the MTHFR gene in North Indian patients with vascular disease. Vascular. 2012 Apr;20(2):88-95.
126. He JA et al. Hyperhomocysteinaemia, low folate concentrations and methylene tetrahydrofolate reductase C677T mutation in acute mesenteric venous thrombosis. Eur J Vasc Endovasc Surg. 2010 Apr;39(4):508-13.
127. Cao H et al. Hyperhomocysteinaemia, low folate concentrations and MTHFR C677T mutation in abdominal aortic aneurysm. Vasa. 2014 May;43(3):181-8.
128. Fekih-Mrissa N et al. Methylenetetrahydrofolate reductase (C677T and A1298C) polymorphisms, hyperhomocysteinemia, and ischemic stroke in Tunisian patients. J Stroke Cerebrovasc Dis. 2013 May;22(4):465-9.
129. Qin X et al. Homocysteine-lowering therapy with folic acid is effective in cardiovascular disease prevention in patients with kidney disease: a meta-analysis of randomized controlled trials. Clin Nutr 2013 Oct;32(5):722-7.
130. Qin X, Wang X et al. Folic acid supplementation with and without vitamin B6 and revascularization risk: a meta-analysis of randomized controlled trials. Clin Nutr 2014 Aug;33(4):603-12.
131. Jason W Locasale. Serine, glycine and the one-carbon cycle: cancer metabolism in full circle. Nat Rev Cancer. Aug 2013; 13(8): 572–583.
132. Mason JB. Folate, cancer risk, and the greek god, Proteus: a tale of two chameleons. Nutr Rev 2009 Apr;67(4):206-12.
133. Duthie SJ. Folate and cancer: how DNA damage, repair and methylation impact on colon carcinogenesis. J Inherit Metab Dis. 2011 Feb;34(1):101-9.
134. Ma Z, Vosseller K. Cancer Metabolism and Elevated O-GlcNAc in Oncogenic Signaling. J Biol Chem 2014 289: 34457-34465.
135. Ebbing M et al. Cancer Incidence and Mortality After Treatment With Folic Acid and Vitamin B12. JAMA. 18 november 2009;302(19):2119-2126.
136. Figueiredo JC et al. Folic acid and risk of prostate cancer: results from a randomized clinical trial. J Natl Cancer Inst 2009 Mar 18;101(6):432-5.
137. Vollset SE et al. Effects of folic acid supplementation on overall and site-specific cancer incidence during the randomised trials: meta-analyses of data on 50,000 individuals. Lancet. 2013 Mar 23;381(9871):1029-36.
138. Qin X et al. Folic acid supplemetation and cancer risk: a meta-analysis of randomized controlled trials. Int J Cancer 2013 Sep 1;133(5):1033-41.
139. Joska TM et al. Regulated DNA Methylation and the Circadian Clock: Implications in Cancer. Biology 2014; 3:560-77.
140. Boumber Y, Issa JP. . Epigenetics in cancer: what's the future? Oncology. 2011;25:220–6, 228.
141. Feinberg AP. Phenotypic plasticity and the epigenetics of human disease. Nature. 2007;447:433–40.
142. Ehrlich M. DNA methylation in cancer: too much, but also too little. Oncogene 2002; 21(35):54000-13.
143. Wong CP et al. Effects of Sulforaphane and 3,3′-Diindolylmethane on Genome-Wide Promoter Methylation in Normal Prostate Epithelial Cells and Prostate Cancer Cells PLoS 2014; DOI: 10.1371/journal.pone.0086787
144. Ho E et al. Dietary Factors and Epigenetic Regulation for Prostate Cancer Prevention. Adv Nutr vol. 2: 497-510, 2011
145. Shorey LE et al. Analysis of genome-wide DNA methylation in CCRF-CEM cells confirms hypermethylation at reported epigenetic markers of T-cell lymphoblastic leukemia and identifies novel therapeutic targets with reduced methylation upon treatment with dietary indoles. Cancer Res April 15, 2012 72; 5430 .
146. Karpf AR, Jones DA. Reactivating the expression of methylation silenced genes in human cancer. Oncogene 2002; 21(35)5496-5503.
147. Sarkar FH. Epigenetics and Cancer. pag 267. Springer Science & Business Media.
148. Krider CS et al. Population red blood cell folate concentrations for prevention of neural tube defects: bayesian model. BMJ 2014; 349
149. Hazra A, Wu K, Kraft P, Fuchs CS, Giovannucci EL, Hunter DJ; ''Twenty-four non-synonymous polymorphisms in the one-carbon metabolic pathway and risk of colorectal adenoma in the Nurses' Health Study.''; Carcinogenesis, 2007
150. Haggarty P, Hoad G, Horgan GW, Campbell DM. DNA methyltransferase candidate polymorphisms, imprinting methylation, and birth outcome. PLoS One. 2013 Jul 26;8(7):e68896.
151. Kono S, Chen K. Genetic polymorphisms of methylenetetrahydrofolate reductase and colorectal cancer and adenoma. Cancer Sci. 2005 Sep;96(9):535-42.
152. Kim DH. The interactive effect of methyl-group diet and polymorphism of methylenetetrahydrofolate reductase on the risk of colorectal cancer. Mutat Res. 2007 Sep 1;622(1-2):14-8.
153. Momb J, Lewandowski JP, Bryant JD, Fitch R, Surman DR, Vokes SA, Appling DR. Deletion of Mthfd1l causes embryonic lethality and neural tube and craniofacial defects in mice. PNAS, 2012; 110 (2): 549
154. Sharp L et al. Polymorphisms in the methylenetetrahydrofolate reductase (MTHFR) gene, intakes of folate and related B vitamins and colorectal cancer: a case-control study in a population with relatively low folate intake. Br J Nutr. 2008 Feb;99(2):379-89.
155. van den Donk M et al. Dietary intake of folate and riboflavin, MTHFR C677T genotype, and colorectal adenoma risk: a Dutch case-control study. Cancer Epidemiol Biomarkers Prev. 2005 Jun;14(6):1562-6.
156. Ohrvik VE, Witthoft CM. Human Folate Bioavailability. Nutrients. 2011 April; 3(4): 475–490.
157. McMahon DM, Liu J, Zhang H, Torres ME, Best RG. Maternal obesity, folate intake, and neural tube defects in offspring. Birth Defects Res A Clin Mol Teratol. 2013 Feb;97(2):115-22.
158. Wang M, Wang ZP, Gao LJ, Gong R, Sun XH, Zhao ZT. Maternal body mass index and the association between folic acid supplements and neural tube defects. Acta Paediatr. 2013 Sep;102(9):908-13.
159. Visentin M et al. The Intestinal Absorption of Folates. Annu Rev Physiol. 2014; 76: 251–274.
160. D. Borradale, E. Isenring, E. Hacker, M.G. Kimlin. Exposure to solar ultraviolet radiation is associated with a decreased folate status in women of childbearing age. J Photochem Photobiol 2014; 131: 90
161. Protiva P et al. Altered folate availability modifies the molecular environment of the human colon: implications for colorectal carcinogenesis. Cancer Prev Res (Phila). 2011 April; 4(4): 530–543.
162. De-Regil LM et al. Effects and safety of periconceptional folate supplementation for preventing birth defects. Cochrane Database Syst Rev. Author manuscript; available in PMC 2014 September 10.
163. Crider KS et al. Folic Acid Food Fortification—Its History, Effect, Concerns, and Future Directions. Nutrients. 2011 March; 3(3): 370–384
164. http://www.voedingonline.nl/page/E-books/Vitamines/Alles-over-Foliumzuur...
165. https://kce.fgov.be/sites/default/files/page_documents/d20041027313.pdf
166. Howerton CL, Bale TL. Targeted placental deletion of OGT recapitulates the prenatal stress phenotype including hypothalamic mitochondrial dysfunction. PNAS 2014; 111(26): 9639–9644
167. Khulan B, Manning JR, Dunbar DR, Seckl JR, Raikkonen K, Eriksson JG, Drake AJ. Epigenomic profiling of men exposed to early-life stress reveals DNA methylation differences in association with current mental state. Transl Psychiatry. 2014 Sep 23;4:e448.
168. Provençal N, Binder EB. The effects of early life stress on the epigenome: From the womb to adulthood and even before. Exp Neurol. 2014 Sep 9.
169. Babenko O, Kovalchuk I, Metz GA. Stress-induced perinatal and transgenerational epigenetic programming of brain development and mental health. Neurosci Biobehav Rev. 2015 Jan;48C:70-91.
170. Desplats PA. Perinatal programming of neurodevelopment: epigenetic mechanisms and the prenatal shaping of the brain. Adv Neurobiol. 2015;10:335-61.
171. Flensborg-Madsen T et al. An association of adult personality with prenatal and early postnatal growth: the EPQ lie-scale. BMC Psychol. 2014 Mar 31;2(1):8.
172. Daskalakis NP, Bagot RC, Parker KJ, Vinkers CH, de Kloet ER. The three-hit concept of vulnerability and resilience: toward understanding adaptation to early-life adversity outcome. Psychoneuroendocrinology. 2013 Sep;38(9):1858-73.
173. Tarry-Adkins JL, Ozanne SE. The impact of early nutrition on the ageing trajectory. Proc Nutr Soc. 2014 May;73(2):289-301.
174. Fall CH et al. Fetal programming and the risk of noncommunicable disease. Indian J Pediatr. 2013 Mar;80 Suppl 1:S13-20
175. Dean SV, Lassi ZS, Imam AM, Bhutta ZA. Preconception care: nutritional risks and interventions. Reprod Health. 2014 Sep 26;11 Suppl 3:S3
176. National Birth Defects Prevention Study. Lack of periconceptional vitamins or supplements that contain folic acid and diabetes mellitus-associated birth defects. Am J Obstet Gynecol. 2012 Mar;206(3):218.e1-13.
177. Wentzel P.Can we prevent diabetic birth defects with micronutrients? Diabetes Obes Metab. 2009 Aug;11(8):770-8.
178. Roy S et al. Effect of maternal micronutrients (folic acid and vitamin B12) and omega 3 fatty acids on indices of brain oxidative stress in the offspring. Brain Dev 2014 Mar;36(3):219-27.
179. Vickers MH. Early life nutrition, epigenetics and programming of later life disease. Nutrients. 2014 Jun 2;6(6):2165-78.
180. Roy S et al. Effect of maternal micronutrients (folic acid and vitamin B12) and omega 3 fatty acids on indices of brain oxidative stress in the offspring. Brain Dev 2014 Mar;36(3):219-27.
181. Sable P et al. Maternal micronutrient imbalance alters gene expression of BDNF, NGF, TrkB and CREB in the offspring brain at an adult age. Int J Dev Neurosci 2014 May;34:24-32.
182. Kemse NG, Kale AA, Joshi SR. Combined Supplementation of Omega-3 Fatty Acids and Micronutrients (Folic Acid, Vitamin B12) Reduces Oxidative Stress Markers in a Rat Model of Pregnancy Induced Hypertension. PLoS One. 2014; 9(11): e111902.
183. Figueiredo JC et al. Vitamins B2, B6 and B12 and Risk of New Colorectal Adenomas in a Randomized Trial of Aspirin Use and Folic Acid Supplementation. Cancer Epidemiol Biomarkers Prev. Aug 2008; 17(8): 2136–2145.
184. Zhang SM et al. Effect of Combined Folic Acid, Vitamin B6, and Vitamin B12 on Cancer Risk in WomenA Randomized Trial. JAMA 2008; 300(17)
185. Ravaglia G et al. Folate, But Not Homocysteine, Predicts the Risk of Fracture in Elderly Persons. J Gerontol A Biol Sci Med Sci (2005) 60 (11): 1458-1462.
186. Zschäbitz S et al. B vitamin intakes and incidence of colorectal cancer: results from the Women's Health Initiative Observational Study cohort. Am J Clin Nutr. Feb 2013; 97(2): 332–343.
Recente studies ondersteunen de rol die B9 en B12 spelen in de pathogenese van AD
187. Sezgin Z, Dincer Y. Alzheimer's disease and epigenetic diet. Neurochem Int. 2014 Dec;78:105-16.
188. Remington R et al. A Phase II Randomized Clinical Trial of a Nutritional Formulation for Cognition and Mood in Alzheimer's Disease. J Alzheimers Dis. 2015 Jan 7. [Epub ahead of print]
189. Otaegui-Arrazola A et al. Diet, cognition, and Alzheimer's disease: food for thought. Eur J Nutr 2014 Feb;53(1):1-23.
190. Remington R et al. A Phase II Randomized Clinical Trial of a Nutritional Formulation for Cognition and Mood in Alzheimer's Disease. J Alzheimers Dis. 2015 Jan 7. [Epub ahead of print]
191. Chen H et al. Associations between Alzheimer's Disease and Blood Homocysteine, Vitamin B12, and Folate: A Case-Control Study. Curr Alzheimer Res. 2015;12(1):88-94.
192. Malek N, Green J. Cognition enhancers for the treatment of dementia. Scott Med J 2014 Nov 27. [Epub ahead of print]
193. Sezgin Z, Dincer Y. Alzheimer's disease and epigenetic diet. Neurochem Int. 2014 Dec;78:105-16.
194. Otaegui-Arrazola A et al. Diet, cognition, and Alzheimer's disease: food for thought. Eur J Nutr 2014 Feb;53(1):1-23.